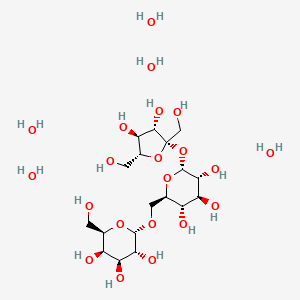
Raffinose
Overview
Description
Raffinose is a trisaccharide composed of galactose, glucose, and fructose. It is naturally found in various plants, including beans, cabbage, brussels sprouts, broccoli, asparagus, and whole grains . This compound plays a significant role in plant stress responses, particularly in temperature sensitivity, seed vigor, resistance to pathogens, and desiccation .
Preparation Methods
Synthetic Routes and Reaction Conditions
Raffinose can be synthesized through enzymatic hydrolysis. The enzyme α-galactosidase hydrolyzes this compound to produce D-galactose and sucrose . The reaction conditions typically involve a pH of approximately 4.5 and a temperature range of 20-25°C .
Industrial Production Methods
In industrial settings, this compound is often extracted from sugar beets or other plant sources. The extraction process involves enzymatic treatment to break down the plant material and release this compound. The enzyme α-galactosidase is commonly used to catalyze the decomposition of this compound, enhancing the yield of sucrose and improving the overall quality of the extracted sugar .
Chemical Reactions Analysis
Types of Reactions
Raffinose undergoes several types of chemical reactions, including hydrolysis, oxidation, and fermentation.
Hydrolysis: This compound can be hydrolyzed by the enzyme α-galactosidase to produce D-galactose and sucrose.
Common Reagents and Conditions
α-Galactosidase: Used for hydrolysis of this compound at pH 4.5 and 20-25°C.
Galactose Dehydrogenase: Used for oxidation of D-galactose to D-galactonic acid.
Major Products
D-Galactose: Produced from the hydrolysis of this compound.
Sucrose: Another product of this compound hydrolysis.
D-Galactonic Acid: Produced from the oxidation of D-galactose.
Scientific Research Applications
Nutritional Applications
Prebiotic Properties:
Raffinose is recognized for its prebiotic potential, promoting the growth of beneficial gut bacteria while inhibiting pathogenic strains. This characteristic is particularly relevant in human health, where it can enhance digestive health and potentially reduce the risk of colorectal cancer by fostering a healthy gut microbiome .
Anti-nutritional Factors:
Despite its benefits, this compound is also considered an anti-nutritional factor due to its association with flatulence in humans and animals. This property limits the consumption of legumes, which are rich in this compound. Understanding these effects is crucial for developing strategies to mitigate flatulence while preserving the nutritional benefits of legumes .
Agricultural Applications
Stress Tolerance in Plants:
this compound plays a critical role in plant stress responses. It helps plants cope with abiotic stresses such as drought, salinity, and extreme temperatures by stabilizing cellular structures and protecting against oxidative damage . For instance, studies on Arabidopsis thaliana have shown that increased levels of this compound correlate with improved tolerance to environmental stressors .
Seed Germination and Vigor:
this compound contributes to seed germination and vigor by acting as a carbohydrate reserve that supports early growth stages under adverse conditions. It regulates physiological processes essential for seed longevity and viability .
Pharmaceutical Applications
Potential Therapeutic Uses:
Research indicates that this compound may have therapeutic properties beyond its nutritional benefits. It has been linked to anti-obesity and anti-diabetic effects, making it a candidate for inclusion in functional foods aimed at managing weight and blood sugar levels . Moreover, its role as an antioxidant suggests potential applications in preventing oxidative stress-related diseases .
Glycobiology Research:
In glycobiology, this compound and its derivatives are studied for their roles in cellular recognition processes. This research could lead to advancements in drug development, particularly in creating glycan-based therapeutics that target specific diseases such as cancer and infectious diseases .
Industrial Applications
Food Industry:
this compound is utilized as a food additive due to its functional properties. It can enhance texture and stability in various food products while also serving as a source of energy for fermentation processes in food production . Its use as a natural sweetener is also being explored.
Cosmetic Industry:
In cosmetics, this compound is valued for its moisturizing properties and potential skin benefits. Its ability to protect skin cells from oxidative damage makes it an attractive ingredient in skincare formulations .
Data Tables
Application Area | Specific Uses | Benefits |
---|---|---|
Nutritional | Prebiotic agent | Promotes gut health |
Anti-nutritional factor | Causes flatulence | |
Agricultural | Stress tolerance | Enhances plant resilience |
Seed vigor | Supports germination | |
Pharmaceutical | Anti-obesity and anti-diabetic | Weight management |
Antioxidant properties | Prevents oxidative stress | |
Industrial | Food additive | Improves texture and stability |
Cosmetic ingredient | Moisturizes skin |
Case Studies
-
Case Study 1: this compound's Role in Gut Health
A study demonstrated that dietary inclusion of this compound significantly increased beneficial bacterial populations in the gut while reducing harmful bacteria. This led to improvements in overall digestive health among participants consuming high-fiber diets rich in legumes . -
Case Study 2: Stress Tolerance Enhancement
Research involving genetically modified Arabidopsis plants showed that overexpression of genes related to this compound synthesis resulted in enhanced tolerance to salinity and chilling stress. These findings suggest potential applications for developing crops resilient to climate change impacts . -
Case Study 3: Glycobiology Insights
A recent investigation into the role of this compound derivatives revealed their potential as therapeutic agents against cancer cells by modulating immune responses through glycan interactions. This highlights the importance of further exploring RFOs in pharmacological research .
Mechanism of Action
Raffinose exerts its effects through various mechanisms:
Prebiotic Effect: This compound is not digestible by humans and monogastric animals, reaching the colon where it stimulates the growth of beneficial gut bacteria such as bifidobacteria and lactobacilli.
Plant Stress Response: In plants, this compound acts as a protective agent during stress conditions by stabilizing cell membranes and proteins, and by scavenging reactive oxygen species.
Comparison with Similar Compounds
Raffinose belongs to the this compound family of oligosaccharides (RFOs), which also includes stachyose and verbascose . These compounds share similar structures and functions but differ in the number of galactose units attached to the sucrose moiety .
Stachyose: A tetrasaccharide composed of two galactose units, one glucose unit, and one fructose unit.
Verbascose: A pentasaccharide composed of three galactose units, one glucose unit, and one fructose unit.
This compound is unique in its specific composition and its role in both plant and human health. It is less complex than stachyose and verbascose, making it easier to study and utilize in various applications .
Biological Activity
Raffinose is a trisaccharide composed of galactose, glucose, and fructose, primarily found in beans, cabbage, broccoli, and whole grains. It belongs to the group of carbohydrates known as this compound family oligosaccharides (RFOs). This article explores the biological activities of this compound, including its metabolic pathways, health implications, and potential therapeutic applications.
Metabolism and Biosynthesis
This compound is synthesized through two primary pathways:
- Galactinol-Dependent Pathway :
- Galactinol-Independent Pathway :
In animals, this compound is not digested due to the absence of the enzyme α-galactosidase. Instead, it passes into the lower gut where it is fermented by gut microbiota, contributing to gas production and digestive discomfort in some individuals .
Biological Functions
This compound exhibits several biological activities that are beneficial to human health:
- Prebiotic Effects : this compound acts as a prebiotic, promoting the growth of beneficial gut bacteria. Approximately 10-15% of gut bacteria can utilize raffinose as a substrate, which may enhance gut health and immune function .
- Antioxidant Properties : Raffinose has been shown to possess antioxidant properties that can help mitigate oxidative stress in cells .
- Anti-inflammatory Effects : Some studies suggest that this compound may exert anti-inflammatory effects, which could be beneficial in managing chronic diseases such as obesity and diabetes .
Health Implications
Despite its benefits, this compound can also have negative effects on health:
- Gastrointestinal Issues : The fermentation of raffinose in the gut can lead to symptoms such as bloating, gas, and abdominal discomfort. This is particularly notable in individuals with irritable bowel syndrome (IBS) or lactose intolerance .
- Potential Allergenicity : Some individuals may experience allergic reactions to foods high in this compound due to their complex carbohydrate structure .
Case Study 1: Prebiotic Potential
A study conducted on individuals consuming diets rich in RFOs demonstrated an increase in beneficial gut microbiota populations. The results indicated that regular consumption of foods containing this compound could improve gut health markers significantly .
Case Study 2: Drought Resistance in Plants
Research on transgenic plants showed that overexpression of this compound synthase enhances drought tolerance through increased synthesis of this compound. This suggests that this compound plays a crucial role in plant stress responses by stabilizing cellular structures under abiotic stress conditions .
Summary Table of Biological Activities
Q & A
Basic Research Questions
Q. What are the established methods for quantifying raffinose in plant tissues, and how do they differ in sensitivity?
- Methodological Answer : this compound quantification typically employs enzymatic assays or high-performance liquid chromatography (HPLC). Enzymatic kits (e.g., hydrolyzing this compound to D-glucose and galactose, followed by spectrophotometric detection) offer cost-effectiveness and simplicity, with sensitivity thresholds of ~0.1 µmol/g . HPLC, using refractive index or evaporative light scattering detectors, provides higher specificity and detects trace amounts (e.g., <0.01 mg/g fresh weight) but requires rigorous sample preparation and calibration . Discrepancies in reported values often stem from extraction protocols (e.g., solvent polarity, tissue homogenization speed) .
Q. What physiological roles does this compound play in plant systems?
- Methodological Answer : this compound functions as a transport sugar in phloem sap and a cryoprotectant during abiotic stress. Studies using isotopic labeling (e.g., C-sucrose tracing) show its accumulation in sink tissues (e.g., seeds) and degradation via α-galactosidase activity in source tissues (e.g., pedicels), facilitating monosaccharide redistribution . Physiological assays in Cucurbitaceae species reveal this compound levels inversely correlate with sucrose concentrations under drought, suggesting osmotic adjustment roles .
Advanced Research Questions
Q. How can researchers investigate the genetic regulation of this compound biosynthesis in plants?
- Methodological Answer : Gene knockout and RNA interference (RNAi) targeting stachyose synthase (STS) or this compound synthase (RAFS) are key approaches. For example, in soybean, STS gene deletion (confirmed via allele-specific PCR markers) reduces stachyose by 90% and increases this compound, highlighting substrate competition in the RFO pathway . Transcriptome profiling (RNA-seq) of mutants under cold stress can identify co-expressed genes (e.g., galactinol synthases), validated via qRT-PCR and enzyme activity assays .
Q. What experimental design considerations are critical when studying this compound accumulation under abiotic stress?
- Methodological Answer : Controlled-environment studies must standardize stress induction (e.g., salinity gradients, temperature ramps) and sampling timepoints. For instance, this compound peaks 24–48 hours after cold exposure in Arabidopsis, requiring hourly sampling to capture dynamics . Confounding factors like diurnal sucrose fluctuations necessitate randomized block designs and normalization to control tissues (e.g., non-stressed leaves from the same node) .
Q. How should conflicting data on this compound levels between studies be analyzed?
- Methodological Answer : Discrepancies often arise from methodological differences. For example, paper chromatography in early studies failed to detect this compound traces (<0.05 mg/g) later quantified via HPLC . Meta-analyses should account for extraction solvents (e.g., 80% ethanol vs. hot water), which differentially solubilize RFOs. Statistical tools like mixed-effects models can adjust for inter-study variability in instrument sensitivity .
Q. What frameworks (e.g., PICO, FINER) are suitable for formulating research questions on this compound metabolism?
- Methodological Answer : The PICO framework (Population, Intervention, Comparison, Outcome) aids in hypothesis-driven studies. For example:
- Population: Zea mays seedlings under hypoxia.
- Intervention: Exogenous this compound supplementation.
- Comparison: Untreated controls.
- Outcome: ATP levels and root elongation rates.
Properties
IUPAC Name |
2-[[6-[3,4-dihydroxy-2,5-bis(hydroxymethyl)oxolan-2-yl]oxy-3,4,5-trihydroxyoxan-2-yl]methoxy]-6-(hydroxymethyl)oxane-3,4,5-triol | |
---|---|---|
Details | Computed by Lexichem TK 2.7.0 (PubChem release 2021.05.07) | |
Source | PubChem | |
URL | https://pubchem.ncbi.nlm.nih.gov | |
Description | Data deposited in or computed by PubChem | |
InChI |
InChI=1S/C18H32O16/c19-1-5-8(22)11(25)13(27)16(31-5)30-3-7-9(23)12(26)14(28)17(32-7)34-18(4-21)15(29)10(24)6(2-20)33-18/h5-17,19-29H,1-4H2 | |
Details | Computed by InChI 1.0.6 (PubChem release 2021.05.07) | |
Source | PubChem | |
URL | https://pubchem.ncbi.nlm.nih.gov | |
Description | Data deposited in or computed by PubChem | |
InChI Key |
MUPFEKGTMRGPLJ-UHFFFAOYSA-N | |
Details | Computed by InChI 1.0.6 (PubChem release 2021.05.07) | |
Source | PubChem | |
URL | https://pubchem.ncbi.nlm.nih.gov | |
Description | Data deposited in or computed by PubChem | |
Canonical SMILES |
C(C1C(C(C(C(O1)OCC2C(C(C(C(O2)OC3(C(C(C(O3)CO)O)O)CO)O)O)O)O)O)O)O | |
Details | Computed by OEChem 2.3.0 (PubChem release 2021.05.07) | |
Source | PubChem | |
URL | https://pubchem.ncbi.nlm.nih.gov | |
Description | Data deposited in or computed by PubChem | |
Molecular Formula |
C18H32O16 | |
Details | Computed by PubChem 2.1 (PubChem release 2021.05.07) | |
Source | PubChem | |
URL | https://pubchem.ncbi.nlm.nih.gov | |
Description | Data deposited in or computed by PubChem | |
DSSTOX Substance ID |
DTXSID50859429 | |
Record name | Hex-2-ulofuranosyl hexopyranosyl-(1->6)hexopyranoside | |
Source | EPA DSSTox | |
URL | https://comptox.epa.gov/dashboard/DTXSID50859429 | |
Description | DSSTox provides a high quality public chemistry resource for supporting improved predictive toxicology. | |
Molecular Weight |
504.4 g/mol | |
Details | Computed by PubChem 2.1 (PubChem release 2021.05.07) | |
Source | PubChem | |
URL | https://pubchem.ncbi.nlm.nih.gov | |
Description | Data deposited in or computed by PubChem | |
CAS No. |
512-69-6 | |
Record name | RAFFINOSE | |
Source | DTP/NCI | |
URL | https://dtp.cancer.gov/dtpstandard/servlet/dwindex?searchtype=NSC&outputformat=html&searchlist=170228 | |
Description | The NCI Development Therapeutics Program (DTP) provides services and resources to the academic and private-sector research communities worldwide to facilitate the discovery and development of new cancer therapeutic agents. | |
Explanation | Unless otherwise indicated, all text within NCI products is free of copyright and may be reused without our permission. Credit the National Cancer Institute as the source. | |
Record name | RAFFINOSE | |
Source | DTP/NCI | |
URL | https://dtp.cancer.gov/dtpstandard/servlet/dwindex?searchtype=NSC&outputformat=html&searchlist=2025 | |
Description | The NCI Development Therapeutics Program (DTP) provides services and resources to the academic and private-sector research communities worldwide to facilitate the discovery and development of new cancer therapeutic agents. | |
Explanation | Unless otherwise indicated, all text within NCI products is free of copyright and may be reused without our permission. Credit the National Cancer Institute as the source. | |
Record name | .alpha.-D-Glucopyranoside, .beta.-D-fructofuranosyl O-.alpha.-D-galactopyranosyl-(1.fwdarw.6)- | |
Source | EPA Chemicals under the TSCA | |
URL | https://www.epa.gov/chemicals-under-tsca | |
Description | EPA Chemicals under the Toxic Substances Control Act (TSCA) collection contains information on chemicals and their regulations under TSCA, including non-confidential content from the TSCA Chemical Substance Inventory and Chemical Data Reporting. | |
Disclaimer and Information on In-Vitro Research Products
Please be aware that all articles and product information presented on BenchChem are intended solely for informational purposes. The products available for purchase on BenchChem are specifically designed for in-vitro studies, which are conducted outside of living organisms. In-vitro studies, derived from the Latin term "in glass," involve experiments performed in controlled laboratory settings using cells or tissues. It is important to note that these products are not categorized as medicines or drugs, and they have not received approval from the FDA for the prevention, treatment, or cure of any medical condition, ailment, or disease. We must emphasize that any form of bodily introduction of these products into humans or animals is strictly prohibited by law. It is essential to adhere to these guidelines to ensure compliance with legal and ethical standards in research and experimentation.