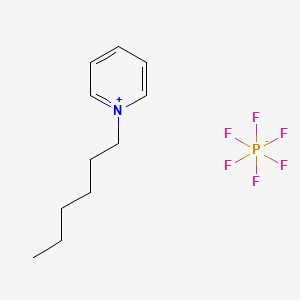
1-Hexylpyridinium hexafluorophosphate
Overview
Description
1-Hexylpyridinium hexafluorophosphate is a pyridinium-based ionic liquid. It is known for its unique properties, such as high thermal stability and low volatility. This compound is often used in various scientific and industrial applications due to its ability to dissolve a wide range of substances and its electrochemical properties .
Mechanism of Action
- Dahi, A., Fatyeyeva, K., Chappey, C., Langevin, D., & Marais, S. (2019). Water molecular state in 1-hexylpyridinium hexafluorophosphate: Water mean cluster size as a function of water concentration. Journal of Molecular Liquids, 292, 111109
- X-MOL. (2019). Water molecular state in this compound
- ChemBK. (Last updated: 2024-04-10). 1-己基吡啶-1-鎓六氟磷酸盐
: Read the full article : Link to abstract : Structure of this compound
Preparation Methods
Synthetic Routes and Reaction Conditions: 1-Hexylpyridinium hexafluorophosphate is typically synthesized through a quaternization reaction. This involves the reaction of pyridine with hexyl bromide to form 1-hexylpyridinium bromide. The resulting compound is then reacted with hexafluorophosphoric acid to yield this compound .
Industrial Production Methods: In an industrial setting, the synthesis of this compound follows similar steps but on a larger scale. The reaction conditions are optimized to ensure high yield and purity. The process involves careful control of temperature, pressure, and reaction time to achieve the desired product .
Chemical Reactions Analysis
Types of Reactions: 1-Hexylpyridinium hexafluorophosphate undergoes various chemical reactions, including:
Substitution Reactions: The hexyl group can be substituted with other alkyl or aryl groups under specific conditions.
Oxidation and Reduction Reactions: The compound can participate in redox reactions, often facilitated by its ionic nature.
Common Reagents and Conditions:
Substitution Reactions: Typically involve reagents such as alkyl halides or aryl halides in the presence of a base.
Oxidation Reactions: Common oxidizing agents include potassium permanganate or hydrogen peroxide.
Reduction Reactions: Reducing agents like sodium borohydride or lithium aluminum hydride are often used.
Major Products: The major products formed from these reactions depend on the specific reagents and conditions used. For example, substitution reactions can yield various alkyl or aryl pyridinium salts, while oxidation and reduction reactions can produce different oxidized or reduced forms of the compound .
Scientific Research Applications
1-Hexylpyridinium hexafluorophosphate has a wide range of applications in scientific research:
Comparison with Similar Compounds
- 1-Hexyl-3-methylimidazolium hexafluorophosphate
- 1-Butyl-3-methylimidazolium tetrafluoroborate
- 1-Hexylpyridinium tetrafluoroborate
Comparison: 1-Hexylpyridinium hexafluorophosphate is unique due to its combination of a pyridinium cation and a hexafluorophosphate anion. This combination provides the compound with distinct properties, such as higher thermal stability and lower volatility compared to similar compounds like 1-hexyl-3-methylimidazolium hexafluorophosphate. Additionally, the pyridinium cation offers different solvation and interaction characteristics compared to imidazolium-based ionic liquids .
Properties
IUPAC Name |
1-hexylpyridin-1-ium;hexafluorophosphate | |
---|---|---|
Source | PubChem | |
URL | https://pubchem.ncbi.nlm.nih.gov | |
Description | Data deposited in or computed by PubChem | |
InChI |
InChI=1S/C11H18N.F6P/c1-2-3-4-6-9-12-10-7-5-8-11-12;1-7(2,3,4,5)6/h5,7-8,10-11H,2-4,6,9H2,1H3;/q+1;-1 | |
Source | PubChem | |
URL | https://pubchem.ncbi.nlm.nih.gov | |
Description | Data deposited in or computed by PubChem | |
InChI Key |
DKRTWJPQQYAIHH-UHFFFAOYSA-N | |
Source | PubChem | |
URL | https://pubchem.ncbi.nlm.nih.gov | |
Description | Data deposited in or computed by PubChem | |
Canonical SMILES |
CCCCCC[N+]1=CC=CC=C1.F[P-](F)(F)(F)(F)F | |
Source | PubChem | |
URL | https://pubchem.ncbi.nlm.nih.gov | |
Description | Data deposited in or computed by PubChem | |
Molecular Formula |
C11H18F6NP | |
Source | PubChem | |
URL | https://pubchem.ncbi.nlm.nih.gov | |
Description | Data deposited in or computed by PubChem | |
DSSTOX Substance ID |
DTXSID7047936 | |
Record name | 1-Hexylpyridinium hexafluorophosphate | |
Source | EPA DSSTox | |
URL | https://comptox.epa.gov/dashboard/DTXSID7047936 | |
Description | DSSTox provides a high quality public chemistry resource for supporting improved predictive toxicology. | |
Molecular Weight |
309.23 g/mol | |
Source | PubChem | |
URL | https://pubchem.ncbi.nlm.nih.gov | |
Description | Data deposited in or computed by PubChem | |
CAS No. |
797789-00-5 | |
Record name | 1-Hexylpyridinium hexafluorophosphate | |
Source | EPA DSSTox | |
URL | https://comptox.epa.gov/dashboard/DTXSID7047936 | |
Description | DSSTox provides a high quality public chemistry resource for supporting improved predictive toxicology. | |
Record name | 1-Hexylpyridinium Hexafluorophosphate | |
Source | European Chemicals Agency (ECHA) | |
URL | https://echa.europa.eu/information-on-chemicals | |
Description | The European Chemicals Agency (ECHA) is an agency of the European Union which is the driving force among regulatory authorities in implementing the EU's groundbreaking chemicals legislation for the benefit of human health and the environment as well as for innovation and competitiveness. | |
Explanation | Use of the information, documents and data from the ECHA website is subject to the terms and conditions of this Legal Notice, and subject to other binding limitations provided for under applicable law, the information, documents and data made available on the ECHA website may be reproduced, distributed and/or used, totally or in part, for non-commercial purposes provided that ECHA is acknowledged as the source: "Source: European Chemicals Agency, http://echa.europa.eu/". Such acknowledgement must be included in each copy of the material. ECHA permits and encourages organisations and individuals to create links to the ECHA website under the following cumulative conditions: Links can only be made to webpages that provide a link to the Legal Notice page. | |
Record name | 1-Hexylpyridinium hexafluorophosphate | |
Source | FDA Global Substance Registration System (GSRS) | |
URL | https://gsrs.ncats.nih.gov/ginas/app/beta/substances/JLW8UBX22E | |
Description | The FDA Global Substance Registration System (GSRS) enables the efficient and accurate exchange of information on what substances are in regulated products. Instead of relying on names, which vary across regulatory domains, countries, and regions, the GSRS knowledge base makes it possible for substances to be defined by standardized, scientific descriptions. | |
Explanation | Unless otherwise noted, the contents of the FDA website (www.fda.gov), both text and graphics, are not copyrighted. They are in the public domain and may be republished, reprinted and otherwise used freely by anyone without the need to obtain permission from FDA. Credit to the U.S. Food and Drug Administration as the source is appreciated but not required. | |
Retrosynthesis Analysis
AI-Powered Synthesis Planning: Our tool employs the Template_relevance Pistachio, Template_relevance Bkms_metabolic, Template_relevance Pistachio_ringbreaker, Template_relevance Reaxys, Template_relevance Reaxys_biocatalysis model, leveraging a vast database of chemical reactions to predict feasible synthetic routes.
One-Step Synthesis Focus: Specifically designed for one-step synthesis, it provides concise and direct routes for your target compounds, streamlining the synthesis process.
Accurate Predictions: Utilizing the extensive PISTACHIO, BKMS_METABOLIC, PISTACHIO_RINGBREAKER, REAXYS, REAXYS_BIOCATALYSIS database, our tool offers high-accuracy predictions, reflecting the latest in chemical research and data.
Strategy Settings
Precursor scoring | Relevance Heuristic |
---|---|
Min. plausibility | 0.01 |
Model | Template_relevance |
Template Set | Pistachio/Bkms_metabolic/Pistachio_ringbreaker/Reaxys/Reaxys_biocatalysis |
Top-N result to add to graph | 6 |
Feasible Synthetic Routes
Disclaimer and Information on In-Vitro Research Products
Please be aware that all articles and product information presented on BenchChem are intended solely for informational purposes. The products available for purchase on BenchChem are specifically designed for in-vitro studies, which are conducted outside of living organisms. In-vitro studies, derived from the Latin term "in glass," involve experiments performed in controlled laboratory settings using cells or tissues. It is important to note that these products are not categorized as medicines or drugs, and they have not received approval from the FDA for the prevention, treatment, or cure of any medical condition, ailment, or disease. We must emphasize that any form of bodily introduction of these products into humans or animals is strictly prohibited by law. It is essential to adhere to these guidelines to ensure compliance with legal and ethical standards in research and experimentation.