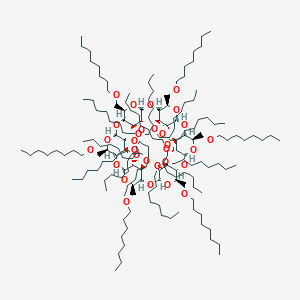
2,3,6-Tri-O-octyl-alpha-cyclodextrin
Overview
Description
2,3,6-Tri-O-octyl-alpha-cyclodextrin is a derivative of alpha-cyclodextrin, a cyclic oligosaccharide composed of six glucose units linked by alpha-1,4-glycosidic bonds. This compound is characterized by the substitution of octyl groups at the 2, 3, and 6 positions of each glucose unit, resulting in a highly hydrophobic molecule. The molecular formula of this compound is C180H348O30, and it has a molecular weight of 2992.67 g/mol .
Preparation Methods
Synthetic Routes and Reaction Conditions: The synthesis of 2,3,6-Tri-O-octyl-alpha-cyclodextrin typically involves the selective alkylation of alpha-cyclodextrin. The process begins with the protection of the hydroxyl groups at the 2 and 3 positions, followed by the selective alkylation of the hydroxyl groups at the 6 position using octyl halides under basic conditions. After deprotection, the hydroxyl groups at the 2 and 3 positions are alkylated with octyl halides to yield the final product .
Industrial Production Methods: Industrial production of this compound follows similar synthetic routes but on a larger scale. The process involves the use of large reactors and optimized reaction conditions to ensure high yield and purity. The product is then purified using techniques such as recrystallization and chromatography .
Chemical Reactions Analysis
Types of Reactions: 2,3,6-Tri-O-octyl-alpha-cyclodextrin primarily undergoes substitution reactions due to the presence of multiple hydroxyl groups. These reactions include nucleophilic substitution and esterification.
Common Reagents and Conditions:
Nucleophilic Substitution: Common reagents include alkyl halides and bases such as sodium hydroxide or potassium carbonate. The reactions are typically carried out in polar aprotic solvents like dimethyl sulfoxide or dimethylformamide.
Esterification: Reagents such as acyl chlorides or anhydrides are used in the presence of a base like pyridine or triethylamine.
Major Products: The major products formed from these reactions are various alkylated and esterified derivatives of this compound, which can be further functionalized for specific applications .
Scientific Research Applications
2,3,6-Tri-O-octyl-alpha-cyclodextrin has a wide range of applications in scientific research due to its unique properties:
Chemistry: It is used as a host molecule in supramolecular chemistry for the formation of inclusion complexes with various guest molecules.
Biology: The compound is used in the study of biological membranes and lipid interactions due to its hydrophobic nature.
Medicine: In drug delivery, this compound is used to enhance the solubility and stability of hydrophobic drugs.
Mechanism of Action
The mechanism of action of 2,3,6-Tri-O-octyl-alpha-cyclodextrin is primarily based on its ability to form inclusion complexes with various guest molecules. The hydrophobic cavity of the cyclodextrin can encapsulate hydrophobic molecules, while the hydrophilic exterior interacts with the aqueous environment. This property is utilized in drug delivery to enhance the solubility and stability of hydrophobic drugs. The molecular targets and pathways involved depend on the specific guest molecule being encapsulated .
Comparison with Similar Compounds
2,3,6-Tri-O-methyl-alpha-cyclodextrin: Similar to 2,3,6-Tri-O-octyl-alpha-cyclodextrin but with methyl groups instead of octyl groups.
2,3,6-Tri-O-benzyl-alpha-cyclodextrin: Contains benzyl groups, making it more hydrophobic than the methyl derivative but less so than the octyl derivative.
Uniqueness: this compound is unique due to its high hydrophobicity, which makes it particularly useful for encapsulating highly hydrophobic molecules. This property distinguishes it from other cyclodextrin derivatives and expands its range of applications in various fields .
Properties
IUPAC Name |
(1R,3R,5R,6R,8R,10R,11R,13R,15R,16R,18R,20R,21R,23R,25R,26R,28R,30R,31S,32R,33S,34R,35S,36R,37S,38R,39S,40R,41S,42R)-31,32,33,34,35,36,37,38,39,40,41,42-dodecaoctoxy-5,10,15,20,25,30-hexakis(octoxymethyl)-2,4,7,9,12,14,17,19,22,24,27,29-dodecaoxaheptacyclo[26.2.2.23,6.28,11.213,16.218,21.223,26]dotetracontane | |
---|---|---|
Source | PubChem | |
URL | https://pubchem.ncbi.nlm.nih.gov | |
Description | Data deposited in or computed by PubChem | |
InChI |
InChI=1S/C180H348O30/c1-19-37-55-73-91-109-127-181-145-151-157-163(187-133-115-97-79-61-43-25-7)169(193-139-121-103-85-67-49-31-13)175(199-151)206-158-152(146-182-128-110-92-74-56-38-20-2)201-177(171(195-141-123-105-87-69-51-33-15)164(158)188-134-116-98-80-62-44-26-8)208-160-154(148-184-130-112-94-76-58-40-22-4)203-179(173(197-143-125-107-89-71-53-35-17)166(160)190-136-118-100-82-64-46-28-10)210-162-156(150-186-132-114-96-78-60-42-24-6)204-180(174(198-144-126-108-90-72-54-36-18)168(162)192-138-120-102-84-66-48-30-12)209-161-155(149-185-131-113-95-77-59-41-23-5)202-178(172(196-142-124-106-88-70-52-34-16)167(161)191-137-119-101-83-65-47-29-11)207-159-153(147-183-129-111-93-75-57-39-21-3)200-176(205-157)170(194-140-122-104-86-68-50-32-14)165(159)189-135-117-99-81-63-45-27-9/h151-180H,19-150H2,1-18H3/t151-,152-,153-,154-,155-,156-,157-,158-,159-,160-,161-,162-,163+,164+,165+,166+,167+,168+,169-,170-,171-,172-,173-,174-,175-,176-,177-,178-,179-,180-/m1/s1 | |
Source | PubChem | |
URL | https://pubchem.ncbi.nlm.nih.gov | |
Description | Data deposited in or computed by PubChem | |
InChI Key |
RMPVMBYXMRFORE-YHIDQUJYSA-N | |
Source | PubChem | |
URL | https://pubchem.ncbi.nlm.nih.gov | |
Description | Data deposited in or computed by PubChem | |
Canonical SMILES |
CCCCCCCCOCC1C2C(C(C(O1)OC3C(OC(C(C3OCCCCCCCC)OCCCCCCCC)OC4C(OC(C(C4OCCCCCCCC)OCCCCCCCC)OC5C(OC(C(C5OCCCCCCCC)OCCCCCCCC)OC6C(OC(C(C6OCCCCCCCC)OCCCCCCCC)OC7C(OC(O2)C(C7OCCCCCCCC)OCCCCCCCC)COCCCCCCCC)COCCCCCCCC)COCCCCCCCC)COCCCCCCCC)COCCCCCCCC)OCCCCCCCC)OCCCCCCCC | |
Source | PubChem | |
URL | https://pubchem.ncbi.nlm.nih.gov | |
Description | Data deposited in or computed by PubChem | |
Isomeric SMILES |
CCCCCCCCOC[C@@H]1[C@@H]2[C@@H]([C@H]([C@H](O1)O[C@@H]3[C@H](O[C@@H]([C@@H]([C@H]3OCCCCCCCC)OCCCCCCCC)O[C@@H]4[C@H](O[C@@H]([C@@H]([C@H]4OCCCCCCCC)OCCCCCCCC)O[C@@H]5[C@H](O[C@@H]([C@@H]([C@H]5OCCCCCCCC)OCCCCCCCC)O[C@@H]6[C@H](O[C@@H]([C@@H]([C@H]6OCCCCCCCC)OCCCCCCCC)O[C@@H]7[C@H](O[C@H](O2)[C@@H]([C@H]7OCCCCCCCC)OCCCCCCCC)COCCCCCCCC)COCCCCCCCC)COCCCCCCCC)COCCCCCCCC)COCCCCCCCC)OCCCCCCCC)OCCCCCCCC | |
Source | PubChem | |
URL | https://pubchem.ncbi.nlm.nih.gov | |
Description | Data deposited in or computed by PubChem | |
Molecular Formula |
C180H348O30 | |
Source | PubChem | |
URL | https://pubchem.ncbi.nlm.nih.gov | |
Description | Data deposited in or computed by PubChem | |
DSSTOX Substance ID |
DTXSID90435201 | |
Record name | 140395-31-9 (name error) | |
Source | EPA DSSTox | |
URL | https://comptox.epa.gov/dashboard/DTXSID90435201 | |
Description | DSSTox provides a high quality public chemistry resource for supporting improved predictive toxicology. | |
Molecular Weight |
2993 g/mol | |
Source | PubChem | |
URL | https://pubchem.ncbi.nlm.nih.gov | |
Description | Data deposited in or computed by PubChem | |
CAS No. |
140395-31-9 | |
Record name | 140395-31-9 (name error) | |
Source | EPA DSSTox | |
URL | https://comptox.epa.gov/dashboard/DTXSID90435201 | |
Description | DSSTox provides a high quality public chemistry resource for supporting improved predictive toxicology. | |
Disclaimer and Information on In-Vitro Research Products
Please be aware that all articles and product information presented on BenchChem are intended solely for informational purposes. The products available for purchase on BenchChem are specifically designed for in-vitro studies, which are conducted outside of living organisms. In-vitro studies, derived from the Latin term "in glass," involve experiments performed in controlled laboratory settings using cells or tissues. It is important to note that these products are not categorized as medicines or drugs, and they have not received approval from the FDA for the prevention, treatment, or cure of any medical condition, ailment, or disease. We must emphasize that any form of bodily introduction of these products into humans or animals is strictly prohibited by law. It is essential to adhere to these guidelines to ensure compliance with legal and ethical standards in research and experimentation.