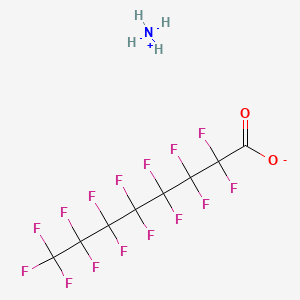
Ammonium perfluorooctanoate
Overview
Description
Ammonium perfluorooctanoate (APFO), also known as Ammonium pentadecafluorooctanoate, is a synthetic compound that has been used extensively in the polymerization of fluorinated monomers . It is the ammonium salt of perfluorooctanoic acid (PFOA) and has been used as a surface-active agent in the polymerisation process for many fluoropolymers . It readily dissociates in water to give the ammonium and perfluorooctanoate ions .
Synthesis Analysis
The synthesis of APFO involves complex chemical processes. One study suggests that APFO undergoes multi-stage pyrolysis including proton transfer, skeletal chain breakage succeeded by a distinct polymerization before C-F bond cleavage .
Molecular Structure Analysis
The molecular formula of APFO is C8H4F15NO2 . It is a completely fluorinated carboxylic acid that is usually used in the ammonium salt form . The structural formula is C7F15COONH4 .
Chemical Reactions Analysis
APFO undergoes multi-stage pyrolysis including proton transfer, skeletal chain breakage succeeded by a distinct polymerization before C-F bond cleavage .
Physical and Chemical Properties Analysis
APFO is a synthetic compound with unique physical and chemical properties. It is used as a processing aid in the production of many fluoropolymers and fluoroelastomers . It readily dissociates in water to give the ammonium and perfluorooctanoate ions .
Scientific Research Applications
1. Analytical Chemistry and Environmental Monitoring
Ammonium perfluorooctanoate (APFO) plays a crucial role in analytical chemistry, particularly in the determination of perfluorooctanoic acid in air samples. A study by Kaiser et al. (2005) developed a method using liquid chromatography-mass spectrometry for measuring perfluorooctanoic acid, a derivative of APFO, in air samples. This method is pivotal for monitoring environmental pollution and occupational safety related to APFO usage (Kaiser et al., 2005).
2. Environmental Degradation and Pollution Control
APFO, as an environmental pollutant, has garnered attention due to its persistence and bioaccumulation. Hao et al. (2014) investigated the decomposition of APFO in aqueous solutions using a combination of persulfate oxidant and ultrasonic irradiation. This study is significant for understanding and developing methods to mitigate APFO pollution in the environment (Hao et al., 2014).
3. Industrial Safety and Health Monitoring
APFO's role in occupational health has been a subject of research. Studies like the one by Sakr et al. (2009) evaluated the mortality risk of ischaemic heart disease among workers exposed to APFO. Such research helps in assessing the safety protocols and health risks in industries using APFO (Sakr et al., 2009).
4. Surface Contamination Assessment
Research by Botelho et al. (2009) focused on developing a wipe test method using liquid chromatography with tandem mass spectrometry for determining PFO, the anion of APFO, on various surfaces. This method aids in assessing the effectiveness of industrial hygiene controls in processes involving APFO products (Botelho et al., 2009).
5. Thermolysis and Material Science
Krusic and Roe (2004) studied the kinetics of thermal decomposition of APFO, which is significant for understanding the stability and decomposition mechanisms of APFO at high temperatures, particularly in fluoropolymer processing (Krusic & Roe, 2004).
6. Wastewater Treatment and Recovery
Xiaofeng et al. (2015) investigated the removal and recovery of PFOA from wastewater using nanofiltration, highlighting the application of APFO in wastewater treatment processes in fluoropolymer production (Xiaofeng et al., 2015).
Mechanism of Action
Target of Action
Ammonium perfluorooctanoate (APFO) primarily targets the peroxisome proliferator-activated receptor alpha (PPARα), a key regulator of lipid metabolism in the liver . It is also known to interact with various proteins involved in lipid homeostasis, such as β- and ω-oxidation enzymes, and diacylglycerol acyltransferases .
Mode of Action
APFO interacts with its targets, particularly PPARα, leading to changes in lipid metabolism. It has been observed that APFO can activate both mouse and human PPARα, albeit in different manners . The activation of PPARα leads to changes in the expression of genes involved in lipid metabolism, which can result in altered lipid profiles .
Biochemical Pathways
APFO affects several biochemical pathways, primarily those involved in lipid metabolism. It has been observed to decrease the production of very low-density lipoprotein (VLDL) and increase VLDL clearance by the liver through increased lipoprotein lipase activity . Additionally, it can lead to decreased cholesteryl ester transfer activity and changes in gene expression of proteins involved in high-density lipoprotein (HDL) metabolism .
Pharmacokinetics
Due to its chemical stability and resistance to degradation, apfo is known to persist in the environment and bioaccumulate in organisms . This persistence and bioaccumulation can impact the bioavailability of APFO, potentially leading to long-term exposure and effects.
Action Environment
Environmental factors can influence the action, efficacy, and stability of APFO. For instance, the presence of other organic compounds, such as urea, can affect the self-assembly of APFO, influencing its interaction with its targets . Additionally, the presence of bacteria capable of degrading APFO can influence its persistence in the environment .
Safety and Hazards
APFO is a confirmed carcinogen and is toxic by inhalation. It is moderately toxic by ingestion and is an eye and skin irritant . It has also been associated with high cholesterol, increased liver enzymes, decreased vaccination response, thyroid disorders, pregnancy-induced hypertension and preeclampsia, and cancer (testicular and kidney) .
Biochemical Analysis
Biochemical Properties
Ammonium perfluorooctanoate plays a crucial role in various biochemical reactions. It interacts with several enzymes, proteins, and other biomolecules. One of the primary interactions is with the peroxisome proliferator-activated receptor alpha (PPARα), which is involved in lipid metabolism. This compound activates PPARα, leading to increased fatty acid oxidation and alterations in lipid profiles . Additionally, it affects the activity of lipoprotein lipase, which is responsible for the hydrolysis of triglycerides in lipoproteins . These interactions highlight the compound’s significant impact on lipid metabolism and energy homeostasis.
Cellular Effects
This compound exerts various effects on different cell types and cellular processes. It has been shown to influence cell signaling pathways, gene expression, and cellular metabolism. For instance, exposure to this compound can lead to hepatomegaly and cellular hypertrophy in liver cells . It also affects the expression of genes involved in lipid metabolism, such as those encoding for enzymes in the β-oxidation pathway . Furthermore, this compound has been associated with disruptions in mitochondrial function and oxidative stress, impacting overall cellular health .
Molecular Mechanism
The molecular mechanism of action of this compound involves several key processes. At the molecular level, it binds to and activates PPARα, leading to changes in gene expression related to lipid metabolism . This activation results in increased β-oxidation of fatty acids and alterations in lipid profiles. Additionally, this compound can inhibit or activate various enzymes involved in lipid and xenobiotic metabolism . These interactions contribute to its overall impact on cellular function and metabolic processes.
Temporal Effects in Laboratory Settings
In laboratory settings, the effects of this compound can change over time. Studies have shown that its stability and degradation can influence its long-term effects on cellular function. For example, prolonged exposure to this compound can lead to persistent alterations in lipid metabolism and liver function . Additionally, its stability in biological systems means that it can accumulate over time, leading to sustained biochemical and cellular effects .
Dosage Effects in Animal Models
The effects of this compound vary with different dosages in animal models. At lower doses, it may not significantly alter plasma lipid levels . At higher doses, it can lead to decreased plasma triglycerides, total cholesterol, and non-high-density lipoprotein cholesterol, while increasing high-density lipoprotein cholesterol . These changes are mediated by alterations in very low-density lipoprotein production and clearance, as well as changes in gene expression related to lipid metabolism . High doses of this compound can also result in toxic effects, such as liver damage and hepatomegaly .
Metabolic Pathways
This compound is involved in several metabolic pathways, particularly those related to lipid metabolism. It activates PPARα, leading to increased β-oxidation of fatty acids and changes in lipid profiles . Additionally, it affects the expression of genes involved in lipid and xenobiotic metabolism, further influencing metabolic flux and metabolite levels . These interactions highlight the compound’s significant impact on overall metabolic processes.
Transport and Distribution
Within cells and tissues, this compound is transported and distributed through various mechanisms. It interacts with transporters and binding proteins, such as organic anion-transporting polypeptide 1A2 and organic anion transporter 4, which mediate its uptake and distribution . These interactions influence its localization and accumulation within specific tissues, such as the liver and kidneys . The compound’s persistence and bioaccumulation potential further contribute to its long-term effects on cellular function and health .
Subcellular Localization
This compound exhibits specific subcellular localization patterns that influence its activity and function. It is primarily localized in the liver, where it affects mitochondrial function and induces peroxisomal proliferation . Additionally, it can interact with various cellular compartments and organelles, leading to changes in cellular metabolism and function . These localization patterns highlight the compound’s targeted effects on specific cellular processes and structures.
Properties
{ "Design of the Synthesis Pathway": "The synthesis of Ammonium perfluorooctanoate can be achieved through a two-step process. The first step involves the synthesis of perfluorooctanoic acid, which is then converted to Ammonium perfluorooctanoate in the second step.", "Starting Materials": [ "Sodium perfluorooctanoate", "Ammonium hydroxide", "Hydrochloric acid", "Sodium hydroxide", "Hydrogen peroxide", "Water" ], "Reaction": [ "Step 1: Synthesis of perfluorooctanoic acid", "1. Dissolve Sodium perfluorooctanoate in water to form a solution.", "2. Add Hydrochloric acid dropwise to the solution while stirring until the pH reaches 2.", "3. Add Hydrogen peroxide to the solution while stirring until the pH reaches 7-8.", "4. Heat the solution to 80-90°C for 2-3 hours.", "5. Cool the solution to room temperature and filter the precipitate.", "6. Wash the precipitate with water and dry it to obtain perfluorooctanoic acid.", "Step 2: Synthesis of Ammonium perfluorooctanoate", "1. Dissolve perfluorooctanoic acid in water to form a solution.", "2. Add Sodium hydroxide to the solution while stirring until the pH reaches 7-8.", "3. Add Ammonium hydroxide to the solution while stirring until the pH reaches 9-10.", "4. Heat the solution to 80-90°C for 2-3 hours.", "5. Cool the solution to room temperature and filter the precipitate.", "6. Wash the precipitate with water and dry it to obtain Ammonium perfluorooctanoate." ] } | |
CAS No. |
3825-26-1 |
Molecular Formula |
C8H4F15NO2 |
Molecular Weight |
431.10 g/mol |
IUPAC Name |
azane;2,2,3,3,4,4,5,5,6,6,7,7,8,8,8-pentadecafluorooctanoic acid |
InChI |
InChI=1S/C8HF15O2.H3N/c9-2(10,1(24)25)3(11,12)4(13,14)5(15,16)6(17,18)7(19,20)8(21,22)23;/h(H,24,25);1H3 |
InChI Key |
YOALFLHFSFEMLP-UHFFFAOYSA-N |
SMILES |
C(=O)(C(C(C(C(C(C(C(F)(F)F)(F)F)(F)F)(F)F)(F)F)(F)F)(F)F)[O-].[NH4+] |
Canonical SMILES |
C(=O)(C(C(C(C(C(C(C(F)(F)F)(F)F)(F)F)(F)F)(F)F)(F)F)(F)F)O.N |
3825-26-1 | |
Pictograms |
Corrosive; Irritant; Health Hazard |
Related CAS |
335-67-1 (Parent) |
Synonyms |
ammonium perfluorooctanoate APFO pentadecafluorooctanoic acid perfluorinated octanoic acid perfluorooctanoate perfluorooctanoic acid perfluorooctanoic acid, anhydride perfluorooctanoic acid, cesium salt perfluorooctanoic acid, chromium (3+) salt perfluorooctanoic acid, cobalt (2+) salt perfluorooctanoic acid, lithium salt perfluorooctanoic acid, monoammonium salt perfluorooctanoic acid, monopotassium salt perfluorooctanoic acid, monosilver (1+) salt perfluorooctanoic acid, monosodium salt perfluorooctanoyl chloride PFOA cpd sodium perfluorooctanoate |
Origin of Product |
United States |
Retrosynthesis Analysis
AI-Powered Synthesis Planning: Our tool employs the Template_relevance Pistachio, Template_relevance Bkms_metabolic, Template_relevance Pistachio_ringbreaker, Template_relevance Reaxys, Template_relevance Reaxys_biocatalysis model, leveraging a vast database of chemical reactions to predict feasible synthetic routes.
One-Step Synthesis Focus: Specifically designed for one-step synthesis, it provides concise and direct routes for your target compounds, streamlining the synthesis process.
Accurate Predictions: Utilizing the extensive PISTACHIO, BKMS_METABOLIC, PISTACHIO_RINGBREAKER, REAXYS, REAXYS_BIOCATALYSIS database, our tool offers high-accuracy predictions, reflecting the latest in chemical research and data.
Strategy Settings
Precursor scoring | Relevance Heuristic |
---|---|
Min. plausibility | 0.01 |
Model | Template_relevance |
Template Set | Pistachio/Bkms_metabolic/Pistachio_ringbreaker/Reaxys/Reaxys_biocatalysis |
Top-N result to add to graph | 6 |
Feasible Synthetic Routes
Disclaimer and Information on In-Vitro Research Products
Please be aware that all articles and product information presented on BenchChem are intended solely for informational purposes. The products available for purchase on BenchChem are specifically designed for in-vitro studies, which are conducted outside of living organisms. In-vitro studies, derived from the Latin term "in glass," involve experiments performed in controlled laboratory settings using cells or tissues. It is important to note that these products are not categorized as medicines or drugs, and they have not received approval from the FDA for the prevention, treatment, or cure of any medical condition, ailment, or disease. We must emphasize that any form of bodily introduction of these products into humans or animals is strictly prohibited by law. It is essential to adhere to these guidelines to ensure compliance with legal and ethical standards in research and experimentation.