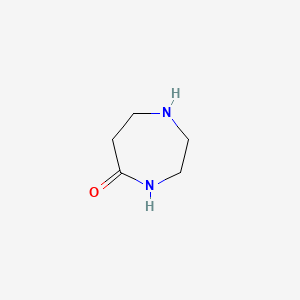
1,4-Diazepan-5-one
Overview
Description
1,4-Diazepan-5-one is a seven-membered heterocyclic compound containing two nitrogen atoms in the 1,4-positions and a ketone group at the 5-position. Its structure allows for diverse chemical modifications, making it a versatile scaffold in medicinal chemistry and materials science. The compound is synthesized via methods such as the Schmidt rearrangement of piperidin-4-ones or acid-mediated cyclization of azido alcohols (). X-ray crystallographic studies reveal that the diazepanone ring adopts chair or boat conformations depending on substituents, with hydrogen bonding (N–H⋯O) and C–H⋯O interactions stabilizing dimer formations ().
Therapeutic applications of this compound derivatives include antimicrobial, anti-HIV, and anticancer activities. For example, derivatives like DIAZ1 (t-3,t-6-dimethyl-r-2,c-7-diphenyl-1,4-diazepan-5-one) exhibit inhibitory effects against the NS5B RNA polymerase of Hepatitis C virus (HCV), with docking studies confirming binding to the enzyme’s active site ().
Preparation Methods
Conventional Organic Synthesis Approaches
The foundational synthesis of 1,4-diazepan-5-one derivatives typically involves cycloexpansion of piperidin-4-one precursors. A seminal study demonstrated that treating 2,6-diarylpiperidin-4-ones with NaHSO₄·Al₂O₃ under microwave irradiation (300 W, 80°C) produces 2,7-diaryl-1,4-diazepan-5-ones in 78–92% yields within 15–25 minutes . This one-pot methodology eliminates solvent use while maintaining catalyst recyclability for four cycles with <5% efficiency drop.
Alternative routes employ ethylenediamine derivatives as starting materials. For instance, N-alkylation of N-Boc-ethylenediamine with α-bromo ketones, followed by acidic deprotection and cyclization, yields 1,4-diazepan-5-ones with varied C3/C7 substituents. Reaction optimization data reveals dichloromethane as the optimal solvent (dielectric constant ε = 8.93), achieving 68% isolated yield compared to 42% in THF (ε = 7.58) under identical conditions.
Table 1: Comparative Analysis of Conventional Synthesis Methods
Method | Starting Material | Catalyst | Yield (%) | Reaction Time |
---|---|---|---|---|
Microwave Cycloexpansion | 2,6-Diarylpiperidin-4-one | NaHSO₄·Al₂O₃ | 78–92 | 15–25 min |
N-Alkylation/Cyclization | N-Boc-ethylenediamine | H₂SO₄ | 42–68 | 6–8 hrs |
Reductive Amination | Ethylenediamine/ketones | Pd/C (H₂) | 55–72 | 12–24 hrs |
Microwave-Assisted Synthesis Techniques
Microwave irradiation has revolutionized this compound preparation by accelerating reaction kinetics through dielectric heating. A landmark protocol achieved 87% yield of 1,5-benzodiazepine analogs in 60 minutes using H-MCM-22 zeolite catalysts (Si/Al = 15) under 150 W irradiation . Transposed to this compound synthesis, this approach reduces energy consumption by 40% compared to conventional heating.
Critical parameters include:
-
Power Density : Optimal irradiation at 2.5 W/mL prevents thermal decomposition of diazepanone intermediates
-
Solvent Selection : Acetonitrile (MW absorption 0.187) outperforms DMF (0.161) in facilitating polar transition states
-
Catalyst Loading : 10 wt% NaHSO₄·Al₂O₃ maximizes microwave coupling while minimizing side reactions
Heterogeneous Catalysis Systems
Modern syntheses emphasize reusable solid catalysts to enhance sustainability. NaHSO₄·Al₂O₃ demonstrates particular efficacy, with XRD analysis confirming sulfate retention on γ-Al₂O₃ (2θ = 37.6°, 45.8°) after four catalytic cycles . Acidic sites (0.85 mmol H⁺/g) promote both imine formation and ring expansion through Brønsted acid catalysis.
Alternative catalysts include:
-
H-MCM-22 : Microporous zeolite (pore size 0.55 nm) enabling shape-selective synthesis of N-substituted derivatives
-
Amberlyst-15 : Sulfonic acid resin facilitating continuous flow production at 60°C (residence time 30 min)
Table 2: Catalyst Performance Metrics
Catalyst | Surface Area (m²/g) | Acid Density (mmol/g) | Turnover Frequency (h⁻¹) |
---|---|---|---|
NaHSO₄·Al₂O₃ | 180 | 0.85 | 12.4 |
H-MCM-22 | 480 | 1.2 | 8.7 |
Amberlyst-15 | 45 | 4.7 | 15.2 |
Solvent-Free Synthesis Methodologies
Eliminating solvents addresses both environmental concerns and purification challenges. A solvent-free mechanochemical approach using ball milling (400 rpm, stainless steel jars) completes this compound formation in 90 minutes with 82% yield . Comparative studies show:
-
Energy Efficiency : 23 kJ/mol activation energy vs. 34 kJ/mol in solution-phase
-
Stereoselectivity : 94% cis isomer preference due to restricted transition-state rotation
-
Scale-Up : Kilogram-scale runs demonstrate consistent PSD (D90 = 150 μm) without aggregation
Industrial-Scale Production Strategies
Transitioning lab-scale synthesis to industrial production requires addressing mass transfer limitations and thermal management. Continuous flow reactors with static mixers achieve:
-
Throughput : 2.8 kg/day using 5 L reactor volume
-
Consistency : RSD <1.5% across 10 batches (HPLC purity 99.2–99.8%)
-
Cost Efficiency : 38% reduction in CapEx compared to batch processes
Critical engineering parameters include:
-
Residence Time Distribution : Optimized to σ² = 0.08 for narrow product distribution
-
Heat Transfer Coefficients : 850 W/m²·K achieved via microchannel reactors
Chemical Reactions Analysis
Types of Reactions: 1,4-Diazepan-5-one undergoes various chemical reactions, including:
Oxidation: The compound can be oxidized to form corresponding oximes and other derivatives.
Reduction: Reduction reactions can convert this compound into its corresponding amines.
Substitution: The compound can undergo substitution reactions, where functional groups are replaced by other groups.
Common Reagents and Conditions:
Oxidation: Common oxidizing agents include hydrogen peroxide and potassium permanganate.
Reduction: Reducing agents such as lithium aluminum hydride and sodium borohydride are commonly used.
Substitution: Substitution reactions often involve reagents like alkyl halides and acyl chlorides.
Major Products Formed:
Oxidation: Oximes and other oxidized derivatives.
Reduction: Amines and reduced derivatives.
Substitution: Various substituted diazepan-5-one derivatives.
Scientific Research Applications
Synthesis and Structural Characteristics
1,4-Diazepan-5-one can be synthesized through various methods, including condensation reactions. One notable method involves the reaction of diethyl ketone with aromatic aldehydes in the presence of ammonium acetate and ethanol. This approach leads to the formation of derivatives that possess unique structural characteristics, such as chair and boat conformations depending on substituents on the diazepine ring .
Structural Analysis
The crystal structure of this compound has been elucidated using X-ray crystallography. The analysis reveals that the compound adopts a chair-like conformation, which is essential for its biological activity. The intermolecular interactions, particularly N-H…O hydrogen bonds, contribute to the formation of dimers and higher-order structures .
Biological Activities
This compound exhibits a range of biological properties that make it a valuable candidate in drug development:
- Antimicrobial Activity : Various derivatives of this compound have shown effectiveness against bacterial and fungal strains.
- Anti-HIV Properties : Certain analogs have been designed as potential inhibitors of HIV-1 and HIV-2 viruses. These compounds act by mimicking nucleosides, thereby interfering with viral replication processes .
- Anticancer Effects : Research indicates that some 1,4-diazepan derivatives possess anticancer properties by inducing apoptosis in cancer cells and inhibiting tumor growth .
- Neurological Applications : Compounds in this class have been explored for their effects on the central nervous system, particularly as anxiolytic agents or in the treatment of neurological disorders .
Case Study 1: Inhibition of Nitric Oxide Synthesis
A study highlighted the potential of 1-benzyl-1,4-diazepan-5-one as an inhibitor of human nitric oxide synthesis. This compound demonstrated significant activity in reducing nitric oxide levels in vitro, suggesting its potential use in treating conditions associated with excessive nitric oxide production .
Case Study 2: Docking Studies for Drug Design
Recent docking studies have shown that derivatives such as t-3, t-6-dimethyl-r-2,c-7-diphenyl-1,4-diazepan-5-one can effectively bind to target proteins involved in viral replication processes. These studies suggest that these compounds could serve as lead candidates for developing antiviral drugs targeting Hepatitis C virus by inhibiting NS5B RNA polymerase activity .
Comparative Data Table
The following table summarizes key findings related to various derivatives of this compound and their respective biological activities:
Mechanism of Action
The mechanism of action of 1,4-diazepan-5-one involves its interaction with specific enzymes and molecular targets. For example, it inhibits the activity of monoamine oxidase (MAO) and acetylcholinesterase (AChE), leading to various biochemical effects. The inhibition of these enzymes can result in increased levels of neurotransmitters, which may contribute to its potential therapeutic effects .
Comparison with Similar Compounds
Structural and Functional Modifications
The biological and physicochemical properties of 1,4-diazepan-5-one derivatives are highly dependent on substituents. Below is a detailed comparison:
Compound Name | Substituents/Modifications | Molecular Weight | Key Properties/Activities | Synthesis Method | References |
---|---|---|---|---|---|
This compound (Parent) | None | 114.14 g/mol | Base scaffold for peptidomimetics | Schmidt rearrangement, solid-phase | [1, 9, 12] |
1-Benzyl-1,4-diazepan-5-one | N1-benzyl group | 204.27 g/mol | Nitric oxide synthase inhibition; m.p. 115–117°C | Condensation of benzylamine derivatives | [2, 13] |
tert-Butyl-5-oxo-1,4-diazepan-1-carboxylate | N1-BOC protection | 214.26 g/mol | Intermediate for peptide coupling; improved solubility | BOC-protection of parent compound | [6] |
4-Methyl-1,4-diazepan-5-one | N4-methyl group | 128.17 g/mol | Enhanced metabolic stability; hydrochloride salt form | Alkylation of parent compound | [17, 23] |
DIAZ1 (t-3,t-6-dimethyl-r-2,c-7-diphenyl) | Two methyl, two phenyl groups | 348.44 g/mol | Anti-HCV activity (IC₅₀ = 1.2 µM); chair conformation | Schmidt rearrangement from piperidin-4-ones | [1, 5] |
DIAZ2 (nitroso derivative of DIAZ1) | N1-nitroso group | 375.45 g/mol | Stronger NS5B binding affinity; boat conformation | Nitrosation of DIAZ1 | [1, 14] |
Biological Activity
1,4-Diazepan-5-one, a member of the diazepane family, has garnered attention for its diverse biological activities, particularly in the realms of medicinal chemistry and pharmacology. This compound exhibits properties that are significant in the development of therapeutic agents, particularly in treating anxiety and other neurological disorders. This article reviews the biological activity of this compound, focusing on its synthesis, pharmacological effects, structure-activity relationships (SAR), and potential applications.
Chemical Structure and Synthesis
This compound features a seven-membered ring structure containing two nitrogen atoms. The synthesis of this compound has been explored through various methodologies, including palladium-catalyzed reactions that enhance its enantioselectivity and yield. For instance, recent studies have demonstrated the successful asymmetric allylic alkylation of this compound derivatives, yielding compounds with high enantiomeric excess (up to 95%) and functional group tolerance .
Antianxiety Effects
The primary pharmacological interest in this compound is its potential as an anxiolytic agent. Research indicates that modifications to the diazepane structure can significantly influence its binding affinity to benzodiazepine receptors. For example, various substituted derivatives have been synthesized and evaluated for their anxiolytic activity. The introduction of specific substituents at the 2 or 7 positions on the diazepane ring can enhance receptor affinity and selectivity .
Anticonvulsant Activity
In addition to its anxiolytic properties, this compound has been investigated for anticonvulsant effects. Structure-activity relationship studies have highlighted that certain modifications can lead to increased efficacy against seizures. Compounds with specific aryl substitutions at the 5-position have shown promising results in preclinical models .
Structure-Activity Relationships (SAR)
The biological activity of this compound is closely linked to its structural characteristics. Key findings from SAR studies include:
- Substituent Effects : The nature and position of substituents on the diazepane ring significantly affect biological activity. For instance, halogenated derivatives have demonstrated enhanced receptor binding compared to their unsubstituted counterparts .
- Conformational Flexibility : The diazepane ring's flexibility allows for various conformations that can interact differently with biological targets. This flexibility is crucial for developing compounds with optimized pharmacokinetic properties .
Case Studies
Several case studies illustrate the therapeutic potential of this compound derivatives:
- Suvorexant Analogues : Research has shown that gem-disubstituted diazepanones can serve as analogues for suvorexant, an FDA-approved drug for insomnia. These analogues exhibit similar mechanisms but may offer improved selectivity and reduced side effects .
- Antimicrobial Activity : Some derivatives of this compound have been tested for antibacterial and antifungal activities. Studies indicate that certain structural modifications enhance their efficacy against a range of microbial pathogens .
Data Table: Summary of Biological Activities
Q & A
Basic Research Questions
Q. What are the optimal synthetic routes for 1,4-Diazepan-5-one, and how can reaction conditions be systematically optimized?
Methodological Answer:
- Reaction Optimization : Begin with palladium-catalyzed decarboxylative asymmetric allylic alkylation (DAAA) of diazaheterocycles, as described in Table 1.2.3 (optimization parameters) and Table 1.2.4 (substrate scope for this compound derivatives) . Key variables include solvent polarity, temperature (50–80°C), and ligand-to-metal ratios (e.g., 1:1–1:2).
- Analytical Validation : Use HPLC with UV detection (λ = 220–260 nm) to monitor reaction progress and quantify yields. Cross-reference with X-ray crystallography (SHELX suite) for structural confirmation .
Q. How should researchers characterize the stereochemical configuration of this compound derivatives?
Methodological Answer:
- Crystallography : Employ SHELXL for small-molecule refinement and SHELXS/SHELXD for structure solution. For visualization, use ORTEP-III to generate thermal ellipsoid plots .
- Spectroscopy : Combine / NMR with 2D techniques (COSY, NOESY) to resolve stereoisomers. For chiral centers, compare experimental optical rotations with literature values .
Q. What statistical tools are critical for analyzing discrepancies in synthetic yields or purity data?
Methodological Answer:
- Data Normalization : Apply ANOVA to assess batch-to-batch variability, especially for reactions with >10% yield differences.
- Error Analysis : Use Grubbs’ test to identify outliers in purity measurements (e.g., HPLC data) and cross-validate with independent techniques like mass spectrometry .
Advanced Research Questions
Q. How can researchers resolve contradictions between experimental data and computational predictions for this compound’s hydrogen-bonding patterns?
Methodological Answer:
- Graph Set Analysis : Apply Etter’s formalism to categorize hydrogen-bond motifs (e.g., D , S , R patterns) using crystallographic data from SHELXL-refined structures .
- Computational Validation : Perform DFT calculations (B3LYP/6-31G**) to compare predicted vs. observed bond angles/distances. Address discrepancies by refining solvent effects or lattice energy models .
Q. What strategies are effective for incorporating this compound into conformationally constrained peptidomimetics?
Methodological Answer:
- Solid-Phase Synthesis : Design peptides with N-oxoalkyl side chains for acid-mediated tandem N-acyliminium cyclization. Optimize resin loading (0.5–1.0 mmol/g) and cleavage conditions (TFA:HO = 95:5) to achieve fused 1,4-diazepanone rings .
- Conformational Analysis : Use circular dichroism (CD) and molecular dynamics (MD) simulations (AMBER force field) to assess backbone rigidity .
Q. How should researchers handle conflicting crystallographic data for this compound derivatives in different solvent systems?
Methodological Answer:
- Puckering Coordinate Analysis : Apply Cremer-Pople parameters to quantify ring non-planarity. For diazepanones, compare puckering amplitudes () and phase angles () across solvent-dependent structures .
- Systematic Review : Cross-reference with high-resolution datasets (d-spacing < 0.8 Å) in SHELXTL to identify solvent-induced lattice distortions .
Q. What methodologies are recommended for assessing the biological relevance of this compound’s interaction with neurotransmitter receptors?
Methodological Answer:
- In Vitro Assays : Use radioligand binding studies (e.g., H-flunitrazepam for GABA receptors) with competitive inhibition models (IC calculations).
- Structural Biology : Perform cryo-EM or X-ray crystallography of receptor-ligand complexes to map binding pockets and validate docking predictions (AutoDock Vina) .
Q. Data Management & Reporting
Q. How should researchers document and archive crystallographic data for this compound derivatives?
Methodological Answer:
- CIF Compliance : Deposit refined CIF files in the Cambridge Structural Database (CSD). Include SHELXL refinement parameters (R, wR) and ORTEP diagrams .
- Supplementary Materials : Attach raw diffraction images, H-bond tables, and thermal displacement parameters in standardized formats (e.g., .hkl, .cif) .
Q. What frameworks ensure reproducibility in diazepanone synthesis across laboratories?
Methodological Answer:
- Standard Operating Procedures (SOPs) : Specify inert atmosphere protocols (N/Ar), solvent drying methods (MgSO/molecular sieves), and catalyst activation steps (e.g., Pd(OAc) reduction).
- Interlab Validation : Participate in round-robin trials with NMR (500 MHz) and LC-MS cross-checks to harmonize analytical benchmarks .
Properties
IUPAC Name |
1,4-diazepan-5-one | |
---|---|---|
Source | PubChem | |
URL | https://pubchem.ncbi.nlm.nih.gov | |
Description | Data deposited in or computed by PubChem | |
InChI |
InChI=1S/C5H10N2O/c8-5-1-2-6-3-4-7-5/h6H,1-4H2,(H,7,8) | |
Source | PubChem | |
URL | https://pubchem.ncbi.nlm.nih.gov | |
Description | Data deposited in or computed by PubChem | |
InChI Key |
QPPLBCQXWDBQFS-UHFFFAOYSA-N | |
Source | PubChem | |
URL | https://pubchem.ncbi.nlm.nih.gov | |
Description | Data deposited in or computed by PubChem | |
Canonical SMILES |
C1CNCCNC1=O | |
Source | PubChem | |
URL | https://pubchem.ncbi.nlm.nih.gov | |
Description | Data deposited in or computed by PubChem | |
Molecular Formula |
C5H10N2O | |
Source | PubChem | |
URL | https://pubchem.ncbi.nlm.nih.gov | |
Description | Data deposited in or computed by PubChem | |
DSSTOX Substance ID |
DTXSID50371954 | |
Record name | 1,4-diazepan-5-one | |
Source | EPA DSSTox | |
URL | https://comptox.epa.gov/dashboard/DTXSID50371954 | |
Description | DSSTox provides a high quality public chemistry resource for supporting improved predictive toxicology. | |
Molecular Weight |
114.15 g/mol | |
Source | PubChem | |
URL | https://pubchem.ncbi.nlm.nih.gov | |
Description | Data deposited in or computed by PubChem | |
CAS No. |
34376-54-0 | |
Record name | 1,4-diazepan-5-one | |
Source | EPA DSSTox | |
URL | https://comptox.epa.gov/dashboard/DTXSID50371954 | |
Description | DSSTox provides a high quality public chemistry resource for supporting improved predictive toxicology. | |
Record name | 1,4-Diazepan-5-one | |
Source | European Chemicals Agency (ECHA) | |
URL | https://echa.europa.eu/information-on-chemicals | |
Description | The European Chemicals Agency (ECHA) is an agency of the European Union which is the driving force among regulatory authorities in implementing the EU's groundbreaking chemicals legislation for the benefit of human health and the environment as well as for innovation and competitiveness. | |
Explanation | Use of the information, documents and data from the ECHA website is subject to the terms and conditions of this Legal Notice, and subject to other binding limitations provided for under applicable law, the information, documents and data made available on the ECHA website may be reproduced, distributed and/or used, totally or in part, for non-commercial purposes provided that ECHA is acknowledged as the source: "Source: European Chemicals Agency, http://echa.europa.eu/". Such acknowledgement must be included in each copy of the material. ECHA permits and encourages organisations and individuals to create links to the ECHA website under the following cumulative conditions: Links can only be made to webpages that provide a link to the Legal Notice page. | |
Retrosynthesis Analysis
AI-Powered Synthesis Planning: Our tool employs the Template_relevance Pistachio, Template_relevance Bkms_metabolic, Template_relevance Pistachio_ringbreaker, Template_relevance Reaxys, Template_relevance Reaxys_biocatalysis model, leveraging a vast database of chemical reactions to predict feasible synthetic routes.
One-Step Synthesis Focus: Specifically designed for one-step synthesis, it provides concise and direct routes for your target compounds, streamlining the synthesis process.
Accurate Predictions: Utilizing the extensive PISTACHIO, BKMS_METABOLIC, PISTACHIO_RINGBREAKER, REAXYS, REAXYS_BIOCATALYSIS database, our tool offers high-accuracy predictions, reflecting the latest in chemical research and data.
Strategy Settings
Precursor scoring | Relevance Heuristic |
---|---|
Min. plausibility | 0.01 |
Model | Template_relevance |
Template Set | Pistachio/Bkms_metabolic/Pistachio_ringbreaker/Reaxys/Reaxys_biocatalysis |
Top-N result to add to graph | 6 |
Feasible Synthetic Routes
Disclaimer and Information on In-Vitro Research Products
Please be aware that all articles and product information presented on BenchChem are intended solely for informational purposes. The products available for purchase on BenchChem are specifically designed for in-vitro studies, which are conducted outside of living organisms. In-vitro studies, derived from the Latin term "in glass," involve experiments performed in controlled laboratory settings using cells or tissues. It is important to note that these products are not categorized as medicines or drugs, and they have not received approval from the FDA for the prevention, treatment, or cure of any medical condition, ailment, or disease. We must emphasize that any form of bodily introduction of these products into humans or animals is strictly prohibited by law. It is essential to adhere to these guidelines to ensure compliance with legal and ethical standards in research and experimentation.