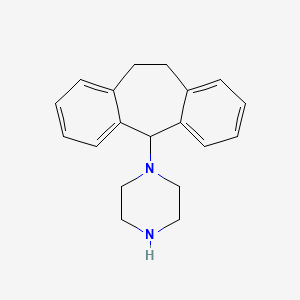
1-(Dibenzosuberyl)piperazine
Overview
Description
1-(Dibenzosuberyl)piperazine is a piperazine derivative featuring a dibenzosuberyl (tricyclic) substituent. Piperazine derivatives are known for diverse applications, including anticancer, psychoactive, and antimicrobial activities, depending on substituent groups .
Preparation Methods
The synthesis of 1-(Dibenzosuberyl)piperazine involves several methods, including:
Cyclization of 1,2-diamine derivatives with sulfonium salts: This method involves the reaction of 1,2-diamine derivatives with sulfonium salts in the presence of a base such as DBU, leading to the formation of protected piperazines.
Ring opening of aziridines: Aziridines can be opened by N-nucleophiles to form piperazine derivatives.
Intermolecular cycloaddition of alkynes: Alkynes bearing amino groups can undergo cycloaddition reactions to form piperazine derivatives.
Chemical Reactions Analysis
1-(Dibenzosuberyl)piperazine undergoes various chemical reactions, including:
Oxidation: This compound can be oxidized using common oxidizing agents such as potassium permanganate or chromium trioxide.
Reduction: Reduction reactions can be carried out using reducing agents like lithium aluminum hydride or sodium borohydride.
Common reagents and conditions used in these reactions include:
Oxidizing agents: Potassium permanganate, chromium trioxide.
Reducing agents: Lithium aluminum hydride, sodium borohydride.
Nucleophiles: Alkyl halides, acyl chlorides.
Major products formed from these reactions include oxidized, reduced, and substituted derivatives of this compound .
Scientific Research Applications
1-(Dibenzosuberyl)piperazine has several scientific research applications, including:
Mechanism of Action
The mechanism of action of 1-(Dibenzosuberyl)piperazine involves its interaction with molecular targets and pathways in biological systems. It is believed to exert its effects by binding to specific receptors or enzymes, leading to changes in cellular functions. The exact molecular targets and pathways involved are still under investigation .
Comparison with Similar Compounds
Comparison with Structural Analogs
Structural Features and Substituent Effects
Piperazine derivatives are characterized by substituents on the nitrogen atoms, which dictate their biological and chemical behavior. Key analogs for comparison include:
- 1-(4-Chlorobenzhydryl)piperazine : Features a diarylmethyl (benzhydryl) group with a chlorine substituent. Exhibits significant cytotoxicity against liver, breast, and colon cancer cell lines (IC₅₀ values in micromolar range) due to cell cycle disruption and apoptosis induction .
- 1-(3-Trifluoromethylphenyl)piperazine (TFMPP) : A psychoactive compound with a trifluoromethylphenyl group. Acts as a serotonin (5-HT) receptor agonist, preferentially targeting 5-HT₁B/₁C receptors, reducing locomotor activity in rodents .
- 1-(3-Chlorophenyl)piperazine (mCPP) : Similar to TFMPP but with a chlorine substituent. Shows serotonergic activity and is metabolized via CYP2D6 to hydroxylated derivatives, contributing to variability in pharmacokinetics .
- 1-(2-Cyanobenzyl)piperazine: Contains an electron-withdrawing cyano group, enhancing solubility and binding in medicinal chemistry applications .
Key Structural Differences :
- Electron-withdrawing groups (e.g., -CF₃ in TFMPP, -CN in 2-cyanobenzyl) enhance binding to serotonin receptors, while bulky groups (e.g., benzhydryl, dibenzosuberyl) may favor anticancer activity by intercalating into cellular structures .
Pharmacological Activities
Table 1: Comparative Pharmacological Profiles
*Inferred based on structural analogs.
Key Observations:
- Anticancer Activity: Bulky substituents like benzhydryl and dibenzosuberyl may enhance cytotoxicity by disrupting cancer cell membranes or DNA synthesis.
- Serotonergic Activity : Smaller substituents (e.g., -CF₃, -Cl) favor 5-HT receptor binding, while larger groups reduce CNS penetration due to increased molecular weight and steric hindrance .
Metabolic and Pharmacokinetic Profiles
- Metabolism : Piperazines undergo N-dealkylation via CYP3A4/2D6, producing active arylpiperazine metabolites. For example, mCPP is metabolized to hydroxylated derivatives, which retain serotonergic activity . The dibenzosuberyl group may slow metabolism due to steric hindrance, prolonging half-life.
- Tissue Distribution : Bulky substituents like dibenzosuberyl could limit blood-brain barrier penetration, reducing psychoactive effects but enhancing peripheral tissue retention .
Biological Activity
1-(Dibenzosuberyl)piperazine is a compound that has garnered interest in the field of medicinal chemistry due to its unique structural characteristics and potential biological activities. This article explores its biological activity, including mechanisms of action, structure-activity relationships (SAR), and relevant case studies.
Chemical Structure
The molecular formula for this compound is C20H20N2, featuring a piperazine ring linked to a dibenzosuberyl group. This structure is significant as both the piperazine moiety and dibenzosuberyl group are known to contribute to various biological activities.
The biological activity of this compound can be attributed to several mechanisms:
- Receptor Interaction : Compounds containing piperazine rings often interact with neurotransmitter receptors, influencing pathways related to mood and cognition.
- Antitumor Activity : Similar piperazine derivatives have shown promising results in inhibiting cancer cell proliferation, suggesting potential applications in oncology.
- Anti-inflammatory Effects : The presence of the dibenzosuberyl group may enhance anti-inflammatory properties, which are common in compounds with similar structures.
Biological Activity Overview
This compound exhibits a range of biological activities, as summarized in the following table:
Activity Type | Description |
---|---|
Antipsychotic | Compounds with piperazine structures often exhibit antipsychotic effects. |
Antitumor | Potential to inhibit the growth of various cancer cell lines. |
Anti-inflammatory | May reduce inflammation through modulation of inflammatory pathways. |
Neuroprotective | Could protect neuronal cells from damage in neurodegenerative conditions. |
Structure-Activity Relationships (SAR)
Research into SAR has indicated that modifications to the piperazine ring or the dibenzosuberyl group can significantly alter biological activity. For instance:
- Substituents on Piperazine : Variations in substituents can enhance receptor binding affinity and selectivity.
- Dibenzosuberyl Modifications : Altering the arrangement or type of substituents on the dibenzosuberyl moiety can influence cytotoxicity and therapeutic efficacy.
Case Studies
Several studies have investigated the biological activity of piperazine derivatives similar to this compound:
- Antitumor Activity Against A549 Cells :
- Neuroprotective Effects :
-
Anti-inflammatory Properties :
- In vitro studies indicated that certain dibenzosuberyl-containing compounds could inhibit pro-inflammatory cytokines, highlighting their potential use in inflammatory disorders.
Future Directions
Given the promising preliminary data on this compound, future research should focus on:
- In Vivo Studies : Assessing efficacy and safety profiles in animal models.
- Mechanistic Studies : Elucidating specific molecular targets and pathways involved in its biological effects.
- Derivatives Exploration : Synthesizing new derivatives to enhance potency and selectivity for specific therapeutic applications.
Q & A
Q. Basic: What are the optimal synthetic routes for 1-(Dibenzosuberyl)piperazine derivatives, and how can reaction conditions be optimized for high yield?
Methodological Answer:
Synthesis of piperazine derivatives often involves nucleophilic substitution or coupling reactions. For example, click chemistry (azide-alkyne cycloaddition) using CuSO₄·5H₂O (0.3 equiv.) and sodium ascorbate (0.6 equiv.) in a H₂O:DCM (1:2) solvent system can yield triazole derivatives efficiently at ambient temperature . Demethylation steps, critical for intermediates, are best achieved using reagents like BBr₃ in dichloromethane, with yields improved by optimizing reaction time (e.g., 6–8 hours) and monitoring via TLC (hexane:ethyl acetate, 1:2) . Key parameters include solvent polarity, catalyst loading, and purification via silica gel chromatography (e.g., ethyl acetate:hexane, 1:8) .
Q. Basic: Which analytical techniques are most effective for distinguishing structural isomers of piperazine derivatives?
Methodological Answer:
Raman microspectroscopy (20 mW laser power, 128–256 scans) combined with multivariate analysis (PCA and LDA) can resolve isomers by highlighting spectral differences in peak position and intensity. For instance, PCA explained 99% variance in trifluoromethylphenyl piperazine isomers using PC4 . Capillary electrophoresis with UV detection (236 nm) and internal standards (e.g., p-tolylpiperazine) separates chlorophenyl isomers (oCPP, mCPP, pCPP) in confiscated samples via optimized buffer pH and voltage .
Q. Advanced: How can multivariate statistical methods like PCA and LDA improve the differentiation of piperazine analogs in spectroscopic data?
Methodological Answer:
PCA reduces dimensionality by identifying principal components (PCs) explaining maximum variance (e.g., PC2–PC4 for benzyl-, chlorophenyl-, and trifluoromethylphenyl-piperazines). LDA then classifies samples using PC scores, achieving >95% separation accuracy. For example, LDA plots differentiated 2-BZP and 1-BZP isomers by leveraging intensity variations at 800–1200 cm⁻¹ Raman shifts . Preprocessing steps (baseline correction, normalization) and parameter optimization (laser power, scan number) are critical for reproducibility.
Q. Advanced: What strategies resolve contradictions in pharmacological data among structurally similar piperazine derivatives?
Methodological Answer:
Systematic structure-activity relationship (SAR) studies are essential. For instance, substituting ethoxy groups from the 3- to 2-position on pyridinylpiperazine alters binding affinities due to steric and electronic effects, as shown in comparative tables . In vitro assays (e.g., receptor-binding studies with radiolabeled ligands) can isolate variables, while molecular docking (e.g., AutoDock Vina) predicts interactions with targets like dopamine receptors . Contradictions in metabolic stability may require HPLC-MS profiling of metabolites under physiological conditions .
Q. Basic: What methodologies ensure accurate quantification of piperazine derivatives in complex matrices like biological samples?
Methodological Answer:
Capillary electrophoresis with UV detection (236 nm) and internal standards (e.g., pTP) achieves selective quantification of TFMPP and mCPP in hair samples, validated via calibration curves (R² > 0.99) and recovery rates (85–110%) . For biological matrices, solid-phase extraction (C18 columns) and LC-MS/MS with multiple reaction monitoring (MRM) enhance sensitivity (LOQ: 0.1 ng/mL) .
Q. Advanced: How do computational chemistry methods contribute to understanding piperazine derivative interactions with biological targets?
Methodological Answer:
Density functional theory (DFT) calculates electrostatic potential surfaces to predict nucleophilic/electrophilic sites, while molecular dynamics simulations (e.g., GROMACS) model binding stability over time. For example, docking studies revealed that 1-(4-Biphenylylcarbonyl)-4-(ethylsulfonyl)piperazine binds to serotonin transporters via hydrophobic interactions with Phe335 and Tyr176 . QSAR models using MOE or Schrödinger Suite correlate substituent logP values with blood-brain barrier permeability .
Q. Basic: What are the critical considerations for ensuring the stability of piperazine derivatives during spectroscopic analysis?
Methodological Answer:
Stability under Raman analysis is confirmed by observing no degradation at 20 mW laser power over 256 scans . For long-term storage, derivatives should be kept in anhydrous DMSO at -20°C, protected from light. Accelerated stability studies (40°C/75% RH for 6 months) with HPLC purity checks (>98%) are recommended for lab-scale batches .
Q. Advanced: How do structural modifications (e.g., substituent position) influence the pharmacokinetics of piperazine derivatives?
Methodological Answer:
Meta-substituted derivatives (e.g., 3-CPP) exhibit higher metabolic stability than para-substituted analogs due to reduced CYP450 oxidation. Ethoxy groups at the 3-position on pyridinylpiperazine enhance aqueous solubility (logS: -2.1 vs. -3.5 for 2-ethoxy) by increasing hydrogen bonding capacity . Pharmacokinetic profiling in rodent models (plasma t₁/₂, AUC) combined with PAMPA assays predicts oral bioavailability differences.
Q. Basic: What chromatographic techniques are recommended for purifying complex piperazine derivatives?
Methodological Answer:
Normal-phase chromatography (silica gel, ethyl acetate:hexane gradients) resolves nonpolar intermediates, while reversed-phase HPLC (C18 column, acetonitrile:water + 0.1% TFA) purifies polar derivatives. For scale-up, isopropanol (>85% v/v) recrystallization of HBr salts achieves >99% purity, as demonstrated for 1-[2-(2,4-dimethylphenylsulfanyl)phenyl]piperazine .
Q. Advanced: What experimental evidence supports the role of specific functional groups in enhancing piperazine bioactivity?
Methodological Answer:
Sulfonyl groups (e.g., in 1-[(2,5-Dimethylthiophen-3-yl)sulfonyl]piperazine) enhance receptor antagonism by forming hydrogen bonds with Thr439 in 5-HT₃ receptors . Nitro groups (e.g., 1-(5-Nitro-thiazol-2-yl)-piperazine) increase antimicrobial potency (MIC: 2 µg/mL vs. S. aureus) by boosting electrophilicity. Functional group replacement studies (e.g., -OCH₃ to -CF₃) quantified via IC50 shifts in kinase inhibition assays .
Properties
IUPAC Name |
1-(2-tricyclo[9.4.0.03,8]pentadeca-1(15),3,5,7,11,13-hexaenyl)piperazine | |
---|---|---|
Source | PubChem | |
URL | https://pubchem.ncbi.nlm.nih.gov | |
Description | Data deposited in or computed by PubChem | |
InChI |
InChI=1S/C19H22N2/c1-3-7-17-15(5-1)9-10-16-6-2-4-8-18(16)19(17)21-13-11-20-12-14-21/h1-8,19-20H,9-14H2 | |
Source | PubChem | |
URL | https://pubchem.ncbi.nlm.nih.gov | |
Description | Data deposited in or computed by PubChem | |
InChI Key |
MDBCLUYDTRHKCA-UHFFFAOYSA-N | |
Source | PubChem | |
URL | https://pubchem.ncbi.nlm.nih.gov | |
Description | Data deposited in or computed by PubChem | |
Canonical SMILES |
C1CC2=CC=CC=C2C(C3=CC=CC=C31)N4CCNCC4 | |
Source | PubChem | |
URL | https://pubchem.ncbi.nlm.nih.gov | |
Description | Data deposited in or computed by PubChem | |
Molecular Formula |
C19H22N2 | |
Source | PubChem | |
URL | https://pubchem.ncbi.nlm.nih.gov | |
Description | Data deposited in or computed by PubChem | |
DSSTOX Substance ID |
DTXSID80219214 | |
Record name | Piperazine, 1-(10,11-dihydrodibenzo(a,d)cyclohepten-5-yl)- | |
Source | EPA DSSTox | |
URL | https://comptox.epa.gov/dashboard/DTXSID80219214 | |
Description | DSSTox provides a high quality public chemistry resource for supporting improved predictive toxicology. | |
Molecular Weight |
278.4 g/mol | |
Source | PubChem | |
URL | https://pubchem.ncbi.nlm.nih.gov | |
Description | Data deposited in or computed by PubChem | |
CAS No. |
69159-50-8 | |
Record name | 1-(10,11-Dihydro-5H-dibenzo[a,d]cyclohepten-5-yl)piperazine | |
Source | CAS Common Chemistry | |
URL | https://commonchemistry.cas.org/detail?cas_rn=69159-50-8 | |
Description | CAS Common Chemistry is an open community resource for accessing chemical information. Nearly 500,000 chemical substances from CAS REGISTRY cover areas of community interest, including common and frequently regulated chemicals, and those relevant to high school and undergraduate chemistry classes. This chemical information, curated by our expert scientists, is provided in alignment with our mission as a division of the American Chemical Society. | |
Explanation | The data from CAS Common Chemistry is provided under a CC-BY-NC 4.0 license, unless otherwise stated. | |
Record name | Piperazine, 1-(10,11-dihydrodibenzo(a,d)cyclohepten-5-yl)- | |
Source | ChemIDplus | |
URL | https://pubchem.ncbi.nlm.nih.gov/substance/?source=chemidplus&sourceid=0069159508 | |
Description | ChemIDplus is a free, web search system that provides access to the structure and nomenclature authority files used for the identification of chemical substances cited in National Library of Medicine (NLM) databases, including the TOXNET system. | |
Record name | Piperazine, 1-(10,11-dihydrodibenzo(a,d)cyclohepten-5-yl)- | |
Source | EPA DSSTox | |
URL | https://comptox.epa.gov/dashboard/DTXSID80219214 | |
Description | DSSTox provides a high quality public chemistry resource for supporting improved predictive toxicology. | |
Record name | 69159-50-8 | |
Source | European Chemicals Agency (ECHA) | |
URL | https://echa.europa.eu/information-on-chemicals | |
Description | The European Chemicals Agency (ECHA) is an agency of the European Union which is the driving force among regulatory authorities in implementing the EU's groundbreaking chemicals legislation for the benefit of human health and the environment as well as for innovation and competitiveness. | |
Explanation | Use of the information, documents and data from the ECHA website is subject to the terms and conditions of this Legal Notice, and subject to other binding limitations provided for under applicable law, the information, documents and data made available on the ECHA website may be reproduced, distributed and/or used, totally or in part, for non-commercial purposes provided that ECHA is acknowledged as the source: "Source: European Chemicals Agency, http://echa.europa.eu/". Such acknowledgement must be included in each copy of the material. ECHA permits and encourages organisations and individuals to create links to the ECHA website under the following cumulative conditions: Links can only be made to webpages that provide a link to the Legal Notice page. | |
Synthesis routes and methods
Procedure details
Disclaimer and Information on In-Vitro Research Products
Please be aware that all articles and product information presented on BenchChem are intended solely for informational purposes. The products available for purchase on BenchChem are specifically designed for in-vitro studies, which are conducted outside of living organisms. In-vitro studies, derived from the Latin term "in glass," involve experiments performed in controlled laboratory settings using cells or tissues. It is important to note that these products are not categorized as medicines or drugs, and they have not received approval from the FDA for the prevention, treatment, or cure of any medical condition, ailment, or disease. We must emphasize that any form of bodily introduction of these products into humans or animals is strictly prohibited by law. It is essential to adhere to these guidelines to ensure compliance with legal and ethical standards in research and experimentation.