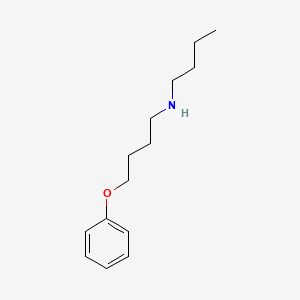
N-butyl-4-phenoxy-1-butanamine
- Click on QUICK INQUIRY to receive a quote from our team of experts.
- With the quality product at a COMPETITIVE price, you can focus more on your research.
Overview
Description
N-Butyl-4-phenoxy-1-butanamine is a secondary amine derivative characterized by a butanamine backbone substituted with a phenoxy group at the 4-position and an n-butyl group on the nitrogen atom. Its molecular formula is C₁₄H₂₃NO, with a molecular weight of 221.34 g/mol. Structurally, it comprises a linear butyl chain attached to the amine nitrogen and a phenoxy moiety (C₆H₅O-) linked to the terminal carbon of the butanamine chain.
Q & A
Basic Research Questions
Q. What are the standard synthetic routes for N-butyl-4-phenoxy-1-butanamine, and how can reaction conditions be optimized to minimize by-products?
The synthesis typically involves nucleophilic substitution or condensation reactions. For example, reacting 4-phenoxy-1-butanol with butylamine under controlled conditions (e.g., reflux in a polar aprotic solvent like acetonitrile) is a common approach. Catalysts such as p-toluenesulfonic acid (PTSA) may accelerate the reaction. Optimization requires monitoring reaction temperature (80–100°C), stoichiometric ratios (1:1.2 alcohol-to-amine), and purification via column chromatography to isolate the product from intermediates like unreacted alcohol or amine .
Q. Which spectroscopic and chromatographic methods are most effective for characterizing this compound, and what key spectral signatures should researchers prioritize?
Nuclear Magnetic Resonance (NMR) spectroscopy is critical for structural confirmation. Key signals include:
- ¹H NMR : A triplet at δ 3.8–4.0 ppm (methylene adjacent to the ether oxygen) and a multiplet at δ 7.2–7.4 ppm (aromatic protons).
- ¹³C NMR : A peak near δ 70 ppm for the ether-linked carbon. Mass spectrometry (MS) should show the molecular ion peak [M+H]⁺ at m/z 235. High-performance liquid chromatography (HPLC) with UV detection (λmax ~228 nm) ensures purity ≥95% .
Advanced Research Questions
Q. How can continuous flow reactors be implemented to improve the scalability and yield of this compound synthesis, and what parameters require optimization?
Continuous flow reactors enhance reproducibility by maintaining precise temperature and mixing control. Key parameters include:
- Residence time : 10–15 minutes for complete conversion.
- Flow rate : 0.5–1.0 mL/min to prevent clogging.
- Catalyst immobilization : Heterogeneous catalysts (e.g., silica-supported PTSA) reduce post-reaction purification. Yields >85% have been reported in flow systems compared to 70–75% in batch reactors .
Q. What strategies are recommended for resolving contradictions in reported biological activity data of this compound across different in vitro models?
Cross-validation using orthogonal assays is essential. For instance:
- Compare results from receptor-binding assays (e.g., serotonin transporter inhibition) with functional cellular assays (e.g., cAMP modulation).
- Control for solvent effects (e.g., DMSO concentrations >0.1% may artifactually enhance membrane permeability).
- Validate findings in primary cell lines to reduce variability from immortalized cell models .
Q. How do electronic and steric effects of substituents on the phenoxy ring influence the compound’s reactivity and interaction with biological targets?
Substituents like chloro (-Cl) or methyl (-CH₃) groups alter electron density and steric bulk:
- Electron-withdrawing groups (e.g., -Cl) : Increase oxidative stability but may reduce nucleophilicity in substitution reactions.
- Steric hindrance : Bulky substituents at the ortho position decrease binding affinity to flat hydrophobic pockets in enzymes. Computational modeling (e.g., DFT for charge distribution) combined with SAR studies can guide rational design .
Comparison with Similar Compounds
Comparison with Structurally Similar Compounds
The following analysis compares N-butyl-4-phenoxy-1-butanamine with three structurally related compounds from the provided evidence, focusing on molecular properties, substituent effects, and inferred physicochemical behaviors.
Structural and Molecular Comparisons
Key Observations:
Substituent Effects on Lipophilicity: The tert-butyl and ethyl groups on the phenoxy ring (e.g., ) increase lipophilicity compared to the unsubstituted phenyl group in this compound. This may enhance membrane permeability but reduce aqueous solubility.
Molecular Weight Trends: Bulkier substituents (e.g., 4-tert-butylphenoxy in ) increase molecular weight significantly (277.45 g/mol vs. 221.34 g/mol for the target compound), which could influence pharmacokinetic properties like diffusion rates.
Structural Diversity: The piperazine-containing compound diverges structurally by replacing the phenoxy group with a 2-methoxyphenyl-piperazine moiety. This introduces hydrogen-bonding capability and polarity, likely altering receptor-binding profiles compared to phenoxy-based analogs.
Preparation Methods
Hydroamination of 1,3-Butadiene as a Foundational Approach
Hydroamination of 1,3-butadiene with primary or secondary amines offers a direct route to unsaturated butenylamines, which can subsequently undergo hydrogenation and phenoxylation to yield N-butyl-4-phenoxy-1-butanamine. Patent US6492558B2 details a two-stage process involving hydroamination followed by transalkylation and hydrogenation .
Catalytic Hydroamination of 1,3-Butadiene
In the first stage, 1,3-butadiene reacts with n-butylamine under hydroaminating conditions. Catalysts such as transition metal complexes (e.g., palladium or nickel) or alkali metal amides facilitate the addition of the amine across the diene system. The reaction typically proceeds at 40–120°C under autogenous pressure (3–30 bar), producing n-butenylamine intermediates like 1-buten-4-ylbutylamine .
Table 1: Hydroamination Reaction Parameters
Parameter | Range | Catalyst Example |
---|---|---|
Temperature | 40–120°C | Pd(OAc)₂ |
Pressure | 3–30 bar | NaNH₂ |
Reaction Time | 2–24 hours | NiCl₂(PPh₃)₂ |
Hydrogenation and Phenoxylation
The unsaturated butenylamine is hydrogenated using heterogeneous catalysts (e.g., Raney nickel or palladium on carbon) under 10–50 bar H₂ at 50–100°C to yield 1-butylbutanamine. Subsequent phenoxylation involves substituting a hydroxyl or halogen group at the fourth carbon with phenoxide. For instance, 4-chloro-1-butylbutanamine reacts with phenol in the presence of a base (e.g., K₂CO₃) in dimethylformamide (DMF) at 80–120°C .
Reductive Amination for N-Alkylation
Reductive amination of ketone precursors with n-butylamine provides an alternative pathway. Patent CN115677544A demonstrates the use of boron trifluoride diethyl etherate (BF₃·Et₂O) and triethylsilane (Et₃SiH) to mediate the reductive coupling of aldehydes with amines .
Synthesis of 4-Oxo-1-butanamine Intermediates
4-Oxo-1-butanamine is prepared via oxidation of 1-butanamine or condensation of γ-butyrolactone with ammonia. The ketone intermediate then undergoes reductive amination with n-butylaldehyde. BF₃·Et₂O activates the carbonyl group, enabling nucleophilic attack by the amine, while Et₃SiH reduces the resulting imine to the secondary amine .
Reaction Scheme:
4-Oxo-1-butanamine+n-ButyraldehydeBF₃\cdotpEt₂O, Et₃SiHN-Butyl-4-oxo-1-butanamineH₂/Pd-CN-Butyl-4-hydroxy-1-butanamine
Phenoxylation of Hydroxyl Intermediates
The hydroxyl group at the fourth carbon is converted to a phenoxy moiety via Mitsunobu reaction or nucleophilic substitution. Using triphenylphosphine (PPh₃) and diethyl azodicarboxylate (DEAD), the hydroxyl group reacts with phenol to form the phenoxy derivative .
Direct introduction of the phenoxy group into pre-formed N-butylbutanamine derivatives is achievable through aromatic substitution. This method is favored when halogenated precursors are accessible.
Synthesis of 4-Chloro-N-butyl-1-butanamine
4-Chloro-N-butyl-1-butanamine is synthesized by chlorinating 1-butanamine at the fourth carbon using SOCl₂ or PCl₅. The chlorine atom is then displaced by phenoxide in a SN2 reaction.
Example Procedure:
-
Chlorination: 1-Butanamine (1.0 equiv) reacts with SOCl₂ (1.2 equiv) in dichloromethane at 0°C for 2 hours.
-
Phenoxylation: The chlorinated intermediate (1.0 equiv) reacts with sodium phenoxide (1.5 equiv) in DMF at 100°C for 12 hours .
Comparative Analysis of Methodologies
Table 2: Advantages and Limitations of Each Method
Properties
Molecular Formula |
C14H23NO |
---|---|
Molecular Weight |
221.34 g/mol |
IUPAC Name |
N-butyl-4-phenoxybutan-1-amine |
InChI |
InChI=1S/C14H23NO/c1-2-3-11-15-12-7-8-13-16-14-9-5-4-6-10-14/h4-6,9-10,15H,2-3,7-8,11-13H2,1H3 |
InChI Key |
MYQAURDYZDFDDI-UHFFFAOYSA-N |
SMILES |
CCCCNCCCCOC1=CC=CC=C1 |
Canonical SMILES |
CCCCNCCCCOC1=CC=CC=C1 |
Origin of Product |
United States |
Retrosynthesis Analysis
AI-Powered Synthesis Planning: Our tool employs the Template_relevance Pistachio, Template_relevance Bkms_metabolic, Template_relevance Pistachio_ringbreaker, Template_relevance Reaxys, Template_relevance Reaxys_biocatalysis model, leveraging a vast database of chemical reactions to predict feasible synthetic routes.
One-Step Synthesis Focus: Specifically designed for one-step synthesis, it provides concise and direct routes for your target compounds, streamlining the synthesis process.
Accurate Predictions: Utilizing the extensive PISTACHIO, BKMS_METABOLIC, PISTACHIO_RINGBREAKER, REAXYS, REAXYS_BIOCATALYSIS database, our tool offers high-accuracy predictions, reflecting the latest in chemical research and data.
Strategy Settings
Precursor scoring | Relevance Heuristic |
---|---|
Min. plausibility | 0.01 |
Model | Template_relevance |
Template Set | Pistachio/Bkms_metabolic/Pistachio_ringbreaker/Reaxys/Reaxys_biocatalysis |
Top-N result to add to graph | 6 |
Feasible Synthetic Routes
Disclaimer and Information on In-Vitro Research Products
Please be aware that all articles and product information presented on BenchChem are intended solely for informational purposes. The products available for purchase on BenchChem are specifically designed for in-vitro studies, which are conducted outside of living organisms. In-vitro studies, derived from the Latin term "in glass," involve experiments performed in controlled laboratory settings using cells or tissues. It is important to note that these products are not categorized as medicines or drugs, and they have not received approval from the FDA for the prevention, treatment, or cure of any medical condition, ailment, or disease. We must emphasize that any form of bodily introduction of these products into humans or animals is strictly prohibited by law. It is essential to adhere to these guidelines to ensure compliance with legal and ethical standards in research and experimentation.