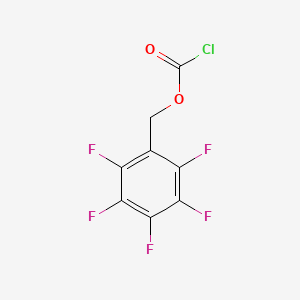
2,3,4,5,6-Pentafluorobenzyl chloroformate
Overview
Description
2,3,4,5,6-Pentafluorobenzyl chloroformate is a fluorinated organic compound with the molecular formula C8H2ClF5O2 and a molecular weight of 260.55 g/mol . It is a derivative of benzyl chloroformate, where the benzene ring is substituted with five fluorine atoms. This compound is primarily used as a reagent in organic synthesis and analytical chemistry.
Mechanism of Action
Target of Action
Pentafluorobenzyl chloroformate, also known as 2,3,4,5,6-Pentafluorobenzyl chloroformate, primarily targets amino acids and alcohols . It is used as a derivatization reagent in the analysis of these compounds .
Mode of Action
The compound interacts with its targets through a process known as derivatization . This process involves the formation of a covalent bond between the target molecule (amino acid or alcohol) and the pentafluorobenzyl group . The reaction proceeds through a nucleophilic substitution, yielding Pentafluorobenzyl chloroformate and sodium bromide as byproducts .
Biochemical Pathways
It is known that the compound plays a crucial role in the detection of amino acids and alcohols in electron capture negative ion chemical ionization mass spectrometry (ecnici-ms) . This suggests that it may influence pathways involving these compounds.
Pharmacokinetics
Its use in analytical chemistry suggests that it may have good stability and reactivity .
Result of Action
The primary result of Pentafluorobenzyl chloroformate’s action is the formation of derivatives of amino acids and alcohols that can be detected using ECNICI-MS . These derivatives exhibit good gas chromatographic properties and provide structurally relevant fragmentation .
Action Environment
The action of Pentafluorobenzyl chloroformate can be influenced by environmental factors such as the presence of a base catalyst and the temperature of the reaction . For instance, the derivatization reaction is promoted by a base catalyst and performed for 30 minutes on a boiling water bath .
Biochemical Analysis
Biochemical Properties
Pentafluorobenzyl chloroformate plays a crucial role in biochemical reactions, primarily as a derivatization reagent. It interacts with various enzymes, proteins, and other biomolecules to form stable derivatives that are easier to detect and analyze. For instance, it reacts with amino acids to form pentafluorobenzyl derivatives, which can be detected with high sensitivity using gas chromatography-mass spectrometry (GC-MS) . This interaction enhances the electron affinity of the amino acids, making them more amenable to detection by electron capture negative ion chemical ionization mass spectrometry (ECNICI-MS) .
Cellular Effects
Pentafluorobenzyl chloroformate influences various cellular processes, including cell signaling pathways, gene expression, and cellular metabolism. It has been shown to affect the derivatization of amino acids and alcohols, which are critical components of cellular metabolism . By modifying these biomolecules, pentafluorobenzyl chloroformate can alter their function and interactions within the cell, potentially impacting cellular signaling pathways and gene expression.
Molecular Mechanism
The molecular mechanism of pentafluorobenzyl chloroformate involves its ability to form covalent bonds with target biomolecules through nucleophilic substitution reactions. This process results in the formation of pentafluorobenzyl derivatives, which exhibit enhanced electron affinity and structural fragmentation in ECNICI-MS . These derivatives are more easily detected and analyzed, providing valuable insights into the molecular composition and interactions of the target biomolecules.
Temporal Effects in Laboratory Settings
In laboratory settings, the effects of pentafluorobenzyl chloroformate can change over time due to its stability and degradation properties. It has been found to be a stable reagent with excellent gas chromatographic properties, making it suitable for long-term studies . Its reactivity and effectiveness may decrease over extended periods, necessitating careful storage and handling to maintain its efficacy.
Dosage Effects in Animal Models
The effects of pentafluorobenzyl chloroformate vary with different dosages in animal models. At lower doses, it can effectively derivatize target biomolecules without causing significant adverse effects. At higher doses, it may exhibit toxic or adverse effects, including potential teratogenicity and developmental toxicity . These effects highlight the importance of optimizing dosage levels to achieve the desired biochemical outcomes while minimizing potential harm.
Metabolic Pathways
Pentafluorobenzyl chloroformate is involved in various metabolic pathways, particularly those related to amino acid metabolism. It interacts with enzymes and cofactors to form stable derivatives that can be traced through metabolic pathways using stable isotope-resolved metabolomics (SIRM) . This approach allows researchers to track the fate of amino acids and other metabolites in cells and tissues, providing valuable insights into metabolic flux and metabolite levels.
Transport and Distribution
Within cells and tissues, pentafluorobenzyl chloroformate is transported and distributed through interactions with transporters and binding proteins. These interactions facilitate its localization and accumulation in specific cellular compartments, where it can exert its biochemical effects . Understanding the transport and distribution mechanisms of pentafluorobenzyl chloroformate is essential for optimizing its use in biochemical research and analysis.
Subcellular Localization
The subcellular localization of pentafluorobenzyl chloroformate is influenced by various targeting signals and post-translational modifications. These factors direct the compound to specific compartments or organelles within the cell, where it can interact with target biomolecules and exert its effects . By understanding the subcellular localization of pentafluorobenzyl chloroformate, researchers can better predict its activity and function in different cellular contexts.
Preparation Methods
2,3,4,5,6-Pentafluorobenzyl chloroformate can be synthesized through various methods. One common synthetic route involves the reaction of 2,3,4,5,6-pentafluorobenzyl alcohol with phosgene (COCl2) in the presence of a base such as pyridine . The reaction typically occurs under anhydrous conditions and at low temperatures to prevent decomposition of the product.
Industrial production methods may involve similar synthetic routes but on a larger scale, with optimized reaction conditions to ensure high yield and purity. The use of automated reactors and continuous flow systems can enhance the efficiency and safety of the production process.
Chemical Reactions Analysis
2,3,4,5,6-Pentafluorobenzyl chloroformate undergoes various chemical reactions, including:
Substitution Reactions: It can react with nucleophiles such as amines, alcohols, and thiols to form corresponding carbamates, esters, and thiocarbamates.
Hydrolysis: In the presence of water, it hydrolyzes to form 2,3,4,5,6-pentafluorobenzyl alcohol and carbon dioxide.
Oxidation and Reduction: While specific oxidation and reduction reactions are less common, the compound can participate in redox reactions under appropriate conditions.
Common reagents used in these reactions include bases like pyridine, nucleophiles such as amines and alcohols, and water for hydrolysis. The major products formed depend on the type of nucleophile and reaction conditions.
Scientific Research Applications
2,3,4,5,6-Pentafluorobenzyl chloroformate has several applications in scientific research:
Analytical Chemistry: It is used as a derivatizing agent for the analysis of various compounds by gas chromatography (GC) and mass spectrometry (MS). The fluorinated derivative enhances the detection sensitivity and selectivity.
Organic Synthesis: It serves as a reagent for the synthesis of fluorinated organic compounds, which are valuable in pharmaceuticals and agrochemicals.
Biological Studies: The compound can be used to modify biomolecules, aiding in the study of enzyme mechanisms and protein interactions.
Industrial Applications: It is employed in the production of specialty chemicals and materials with unique properties, such as high thermal stability and resistance to chemical degradation.
Comparison with Similar Compounds
2,3,4,5,6-Pentafluorobenzyl chloroformate can be compared with other similar compounds such as:
2,3,4,5,6-Pentafluorobenzoyl chloride: Another fluorinated reagent used in organic synthesis and analytical chemistry.
4-Nitrobenzyl chloroformate: Used for similar derivatization purposes but lacks the fluorine atoms, resulting in different reactivity and properties.
2,3,4,5,6-Pentafluorobenzyl bromide: Similar structure but with a bromine atom instead of a chloroformate group, used in different types of reactions.
The uniqueness of this compound lies in its combination of a highly electrophilic chloroformate group and the electron-withdrawing effects of the fluorine atoms, which enhance its reactivity and stability in various applications.
Properties
IUPAC Name |
(2,3,4,5,6-pentafluorophenyl)methyl carbonochloridate | |
---|---|---|
Source | PubChem | |
URL | https://pubchem.ncbi.nlm.nih.gov | |
Description | Data deposited in or computed by PubChem | |
InChI |
InChI=1S/C8H2ClF5O2/c9-8(15)16-1-2-3(10)5(12)7(14)6(13)4(2)11/h1H2 | |
Source | PubChem | |
URL | https://pubchem.ncbi.nlm.nih.gov | |
Description | Data deposited in or computed by PubChem | |
InChI Key |
CAKTVARYLWEKSA-UHFFFAOYSA-N | |
Source | PubChem | |
URL | https://pubchem.ncbi.nlm.nih.gov | |
Description | Data deposited in or computed by PubChem | |
Canonical SMILES |
C(C1=C(C(=C(C(=C1F)F)F)F)F)OC(=O)Cl | |
Source | PubChem | |
URL | https://pubchem.ncbi.nlm.nih.gov | |
Description | Data deposited in or computed by PubChem | |
Molecular Formula |
C8H2ClF5O2 | |
Source | PubChem | |
URL | https://pubchem.ncbi.nlm.nih.gov | |
Description | Data deposited in or computed by PubChem | |
DSSTOX Substance ID |
DTXSID30201740 | |
Record name | Pentafluorobenzyl chloroformate | |
Source | EPA DSSTox | |
URL | https://comptox.epa.gov/dashboard/DTXSID30201740 | |
Description | DSSTox provides a high quality public chemistry resource for supporting improved predictive toxicology. | |
Molecular Weight |
260.54 g/mol | |
Source | PubChem | |
URL | https://pubchem.ncbi.nlm.nih.gov | |
Description | Data deposited in or computed by PubChem | |
CAS No. |
53526-74-2 | |
Record name | Pentafluorobenzyl chloroformate | |
Source | ChemIDplus | |
URL | https://pubchem.ncbi.nlm.nih.gov/substance/?source=chemidplus&sourceid=0053526742 | |
Description | ChemIDplus is a free, web search system that provides access to the structure and nomenclature authority files used for the identification of chemical substances cited in National Library of Medicine (NLM) databases, including the TOXNET system. | |
Record name | Pentafluorobenzyl chloroformate | |
Source | EPA DSSTox | |
URL | https://comptox.epa.gov/dashboard/DTXSID30201740 | |
Description | DSSTox provides a high quality public chemistry resource for supporting improved predictive toxicology. | |
Retrosynthesis Analysis
AI-Powered Synthesis Planning: Our tool employs the Template_relevance Pistachio, Template_relevance Bkms_metabolic, Template_relevance Pistachio_ringbreaker, Template_relevance Reaxys, Template_relevance Reaxys_biocatalysis model, leveraging a vast database of chemical reactions to predict feasible synthetic routes.
One-Step Synthesis Focus: Specifically designed for one-step synthesis, it provides concise and direct routes for your target compounds, streamlining the synthesis process.
Accurate Predictions: Utilizing the extensive PISTACHIO, BKMS_METABOLIC, PISTACHIO_RINGBREAKER, REAXYS, REAXYS_BIOCATALYSIS database, our tool offers high-accuracy predictions, reflecting the latest in chemical research and data.
Strategy Settings
Precursor scoring | Relevance Heuristic |
---|---|
Min. plausibility | 0.01 |
Model | Template_relevance |
Template Set | Pistachio/Bkms_metabolic/Pistachio_ringbreaker/Reaxys/Reaxys_biocatalysis |
Top-N result to add to graph | 6 |
Feasible Synthetic Routes
Disclaimer and Information on In-Vitro Research Products
Please be aware that all articles and product information presented on BenchChem are intended solely for informational purposes. The products available for purchase on BenchChem are specifically designed for in-vitro studies, which are conducted outside of living organisms. In-vitro studies, derived from the Latin term "in glass," involve experiments performed in controlled laboratory settings using cells or tissues. It is important to note that these products are not categorized as medicines or drugs, and they have not received approval from the FDA for the prevention, treatment, or cure of any medical condition, ailment, or disease. We must emphasize that any form of bodily introduction of these products into humans or animals is strictly prohibited by law. It is essential to adhere to these guidelines to ensure compliance with legal and ethical standards in research and experimentation.