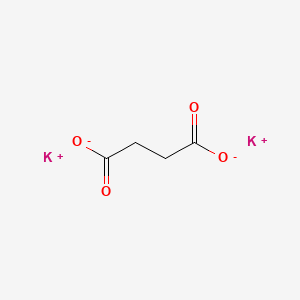
Potassium Succinate
Overview
Description
Mechanism of Action
Target of Action
Dipotassium succinate, also known as this compound, primarily targets the tricarboxylic acid (TCA) cycle in the mitochondria . It serves as a substrate for succinate dehydrogenase (SDH) , an enzyme that plays a crucial role in energy production within fundamental mitochondrial metabolic pathways .
Mode of Action
Once inside the cell, dithis compound participates in the TCA cycle, where it is converted to fumarate by SDH . This reaction contributes to the production of ATP, the primary energy currency of the cell . The compound’s interaction with its target (SDH) leads to changes in energy production and other downstream effects.
Biochemical Pathways
Dithis compound is involved in the TCA cycle, a central metabolic pathway in cells . It is converted to fumarate by SDH, contributing to the production of ATP . Additionally, succinate and SDH are involved in various metabolic pathways, contributing to the regulation of numerous catabolic and anabolic processes .
Pharmacokinetics
It is known that succinate can be rapidly metabolized in the tca cycle once it enters the body .
Result of Action
The action of dithis compound results in energy production and the regulation of various metabolic processes . Elevated levels of succinate have been associated with pathological states, including chronic inflammation, ischemia/reperfusion (IR) injury, and cancer . These associations are primarily due to exaggerated immune cell responses .
Action Environment
The action of dithis compound can be influenced by various environmental factors. For instance, under hypoxic conditions common in inflammatory environments, disruptions in the TCA cycle lead to cytoplasmic succinate accumulation . This can contribute to several biological processes such as stabilization of hypoxia-inducible factor-1α (HIF-1α), generation of reactive oxygen species (ROS), and protein succinylation .
Biochemical Analysis
Biochemical Properties
Dipotassium succinate plays a crucial role in biochemical reactions, particularly in the citric acid cycle (Krebs cycle). It acts as an intermediate in the cycle, facilitating the conversion of succinyl-CoA to succinate. This compound interacts with several enzymes, including succinate dehydrogenase, which catalyzes the oxidation of succinate to fumarate. Additionally, dithis compound can interact with proteins and other biomolecules, influencing various metabolic processes .
Cellular Effects
Dithis compound has significant effects on various types of cells and cellular processes. It influences cell function by participating in the citric acid cycle, which is essential for cellular respiration and energy production. The compound can impact cell signaling pathways, gene expression, and cellular metabolism. For instance, it can modulate the activity of succinate dehydrogenase, affecting the production of ATP and other metabolites .
Molecular Mechanism
At the molecular level, dithis compound exerts its effects through binding interactions with biomolecules. It acts as a substrate for succinate dehydrogenase, facilitating the enzyme’s catalytic activity. This interaction leads to the oxidation of succinate to fumarate, a key step in the citric acid cycle. Additionally, dithis compound can influence gene expression by modulating the activity of transcription factors and other regulatory proteins .
Temporal Effects in Laboratory Settings
In laboratory settings, the effects of dithis compound can change over time. The compound’s stability and degradation can influence its long-term effects on cellular function. Studies have shown that dithis compound remains stable under standard laboratory conditions, but its activity may decrease over extended periods. Long-term exposure to dithis compound can lead to changes in cellular metabolism and function, which are important considerations for in vitro and in vivo studies .
Dosage Effects in Animal Models
The effects of dithis compound can vary with different dosages in animal models. At low doses, the compound can enhance metabolic activity and energy production. At high doses, it may exhibit toxic or adverse effects, such as disrupting cellular homeostasis and causing oxidative stress. Threshold effects have been observed, indicating that there is a specific dosage range within which dithis compound is beneficial without causing harm .
Metabolic Pathways
Dithis compound is involved in several metabolic pathways, including the citric acid cycle and the glyoxylate cycle. It interacts with enzymes such as succinate dehydrogenase and fumarase, influencing the flux of metabolites through these pathways. The compound can also affect the levels of other metabolites, such as fumarate and malate, by modulating the activity of key enzymes .
Transport and Distribution
Within cells and tissues, dithis compound is transported and distributed through various mechanisms. It can be taken up by cells via specific transporters and distributed to different cellular compartments. The compound may interact with binding proteins that facilitate its transport and localization. Additionally, dithis compound can accumulate in certain tissues, influencing its overall bioavailability and activity .
Subcellular Localization
Dithis compound is localized in specific subcellular compartments, such as the mitochondria, where it participates in the citric acid cycle. The compound’s activity and function can be influenced by its subcellular localization. For example, targeting signals and post-translational modifications can direct dithis compound to the mitochondria, enhancing its role in energy production and metabolic regulation .
Preparation Methods
Synthetic Routes and Reaction Conditions
Potassium Succinate can be synthesized through the neutralization reaction between succinic acid and potassium hydroxide. The reaction is typically carried out in an aqueous solution, where succinic acid (C4H6O4) reacts with potassium hydroxide (KOH) to form dithis compound (C4H4K2O4) and water (H2O):
C4H6O4 + 2KOH → C4H4K2O4 + 2H2O
Industrial Production Methods
In industrial settings, dithis compound is produced by reacting succinic acid with potassium carbonate (K2CO3) or potassium bicarbonate (KHCO3). The reaction is conducted in a controlled environment to ensure the complete conversion of reactants to the desired product. The resulting dithis compound is then purified through crystallization and drying processes .
Chemical Reactions Analysis
Types of Reactions
Potassium Succinate undergoes various chemical reactions, including:
Oxidation: It can be oxidized to form potassium fumarate.
Reduction: It can be reduced to form this compound.
Substitution: It can participate in substitution reactions with other chemical reagents.
Common Reagents and Conditions
Oxidation: Potassium permanganate (KMnO4) is commonly used as an oxidizing agent.
Reduction: Hydrogen gas (H2) in the presence of a catalyst such as palladium on carbon (Pd/C) is used for reduction.
Substitution: Various halogenating agents can be used for substitution reactions.
Major Products Formed
Oxidation: Potassium fumarate (C4H2K2O4)
Reduction: this compound (C4H6K2O4)
Substitution: Halogenated derivatives of succinic acid
Scientific Research Applications
Potassium Succinate has a wide range of applications in scientific research, including:
Chemistry: It is used as a buffering agent in various chemical reactions and analytical procedures.
Biology: It serves as a metabolic intermediate in the citric acid cycle, playing a crucial role in cellular respiration.
Medicine: It is used in the formulation of certain pharmaceutical products due to its buffering properties.
Industry: It is employed in the production of food additives, cosmetics, and other consumer products
Comparison with Similar Compounds
Similar Compounds
- Potassium succinate (C4H5KO4)
- Sodium succinate (C4H4Na2O4)
- Ammonium succinate (C4H8N2O4)
Uniqueness
This compound is unique due to its specific potassium content, which imparts distinct buffering properties and solubility characteristics. Compared to sodium succinate, it has a higher solubility in water and a different pH buffering range. Its potassium ions also play a vital role in various biological processes, making it particularly useful in medical and biochemical applications .
Properties
CAS No. |
676-47-1 |
---|---|
Molecular Formula |
C4H6KO4 |
Molecular Weight |
157.19 g/mol |
IUPAC Name |
dipotassium;butanedioate |
InChI |
InChI=1S/C4H6O4.K/c5-3(6)1-2-4(7)8;/h1-2H2,(H,5,6)(H,7,8); |
InChI Key |
ZCWYDQNATAJCIN-UHFFFAOYSA-N |
SMILES |
C(CC(=O)[O-])C(=O)[O-].[K+].[K+] |
Canonical SMILES |
C(CC(=O)O)C(=O)O.[K] |
676-47-1 22445-04-1 |
|
Pictograms |
Irritant |
Synonyms |
1,2 Ethanedicarboxylic Acid 1,2-Ethanedicarboxylic Acid 1,4 Butanedioic Acid 1,4-Butanedioic Acid Ammonium Succinate Butanedioic Acid Potassium Succinate Succinate Succinate, Ammonium Succinate, Potassium Succinic Acid |
Origin of Product |
United States |
Synthesis routes and methods
Procedure details
Retrosynthesis Analysis
AI-Powered Synthesis Planning: Our tool employs the Template_relevance Pistachio, Template_relevance Bkms_metabolic, Template_relevance Pistachio_ringbreaker, Template_relevance Reaxys, Template_relevance Reaxys_biocatalysis model, leveraging a vast database of chemical reactions to predict feasible synthetic routes.
One-Step Synthesis Focus: Specifically designed for one-step synthesis, it provides concise and direct routes for your target compounds, streamlining the synthesis process.
Accurate Predictions: Utilizing the extensive PISTACHIO, BKMS_METABOLIC, PISTACHIO_RINGBREAKER, REAXYS, REAXYS_BIOCATALYSIS database, our tool offers high-accuracy predictions, reflecting the latest in chemical research and data.
Strategy Settings
Precursor scoring | Relevance Heuristic |
---|---|
Min. plausibility | 0.01 |
Model | Template_relevance |
Template Set | Pistachio/Bkms_metabolic/Pistachio_ringbreaker/Reaxys/Reaxys_biocatalysis |
Top-N result to add to graph | 6 |
Feasible Synthetic Routes
Q1: What is the molecular formula and weight of potassium succinate?
A1: The molecular formula for this compound is C4H4K2O4. Its molecular weight is 248.32 g/mol. [, ]
Q2: Is there spectroscopic data available for this compound?
A2: Yes, researchers have used techniques like Fourier-transform infrared (FT-IR) and FT-Raman spectroscopy to characterize this compound. These analyses provide insights into the vibrational modes and chemical bonds within the molecule. []
Q3: Does the crystal structure of this compound influence its properties?
A3: Yes, this compound trihydrate (C4H4O4K2·3H2O) crystallizes in an orthorhombic system. This structure, where succinate ions and water molecules lie on mirror planes, is stabilized by hydrogen bonds and interactions with potassium ions. These structural features likely contribute to its observed properties. [, ]
Q4: Has this compound shown promise in specific material applications?
A4: Research indicates that this compound can be used as a compatibilizer to improve the dispersion of clay layers within a polypropylene matrix. This has implications for enhancing the mechanical and physical properties of the resulting composite materials. []
Q5: What is known about the catalytic properties of this compound?
A5: this compound plays a role in biochemical reactions. For instance, it can be used as a respiratory substrate in studies examining oxidative phosphorylation and proton exchange in isolated mitochondria. [, ]
Q6: Have computational methods been applied to study this compound?
A6: Yes, molecular docking studies have been employed to investigate the potential antibacterial action of this compound-succinic acid. These simulations help to visualize and predict how the compound might interact with bacterial targets. []
Q7: How stable is this compound under different conditions?
A7: While specific stability data might vary depending on the formulation and storage conditions, this compound is generally considered stable. It is crucial to consider factors like temperature, humidity, and exposure to light when formulating and storing this compound. [, , ]
Q8: Does this compound have any known biological effects?
A8: Research suggests potential roles for this compound in several biological processes:
- Cardioprotective Effects: Some studies suggest this compound may offer cardioprotective benefits, particularly in models of myocardial infarction. This potential benefit is thought to be linked to its influence on cellular energy metabolism. [, , ]
- Influence on Renal Function: this compound has been shown to impact renal function, particularly in relation to diuresis and electrolyte balance. Studies in rats demonstrate that potassium loading, including with this compound, can increase glomerular filtration rate and influence the excretion of sodium, potassium, and chloride. [, ]
- Impact on Plant Growth: this compound has been explored as a potential growth regulator in plant tissue culture. Studies suggest it may positively influence the regeneration and growth of microshoots in certain plant species. []
Q9: Are there any known interactions of this compound with enzymes?
A9: this compound has been found to influence the activity of certain enzymes:
- Phosphofructokinase: this compound can activate phosphofructokinase, a key regulatory enzyme in glycolysis, in carrot roots. This activation is thought to be primarily due to the succinate anion. Understanding these interactions is important for comprehending the regulation of carbohydrate metabolism in plants. []
- Adenosine 5'-Monophosphate Aminohydrolase: The activity of this enzyme, involved in nucleotide metabolism, is influenced by the presence of this compound. Studies on cod muscle extracts have shown that this compound can affect the enzyme's activity levels under specific conditions. []
Q10: What is known about the toxicity profile of this compound?
A10: While generally considered safe for its intended uses, limited data is available on the long-term toxicity of this compound. As with any chemical compound, it's crucial to handle it with care and follow appropriate safety guidelines. [, ]
Q11: Is there information available regarding the environmental impact of this compound?
A11: Research on the ecotoxicological effects of this compound is scarce. Given the growing emphasis on sustainability, it's essential to investigate its potential impact on the environment and explore strategies to mitigate any negative effects. []
Disclaimer and Information on In-Vitro Research Products
Please be aware that all articles and product information presented on BenchChem are intended solely for informational purposes. The products available for purchase on BenchChem are specifically designed for in-vitro studies, which are conducted outside of living organisms. In-vitro studies, derived from the Latin term "in glass," involve experiments performed in controlled laboratory settings using cells or tissues. It is important to note that these products are not categorized as medicines or drugs, and they have not received approval from the FDA for the prevention, treatment, or cure of any medical condition, ailment, or disease. We must emphasize that any form of bodily introduction of these products into humans or animals is strictly prohibited by law. It is essential to adhere to these guidelines to ensure compliance with legal and ethical standards in research and experimentation.