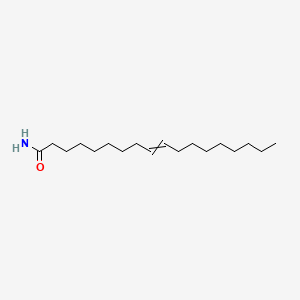
9-Octadecenamide
Overview
Description
9-Octadecenamide: oleamide or oleic acid amide , is an organic compound with the formula C18H35NO . It is a fatty acid amide derived from oleic acid and is commonly found as a white crystalline or waxy solid. This compound is known for its role as a bioactive molecule and is used in various industrial applications due to its lubricating and anti-static properties .
Scientific Research Applications
Chemistry: 9-Octadecenamide is used as a slip agent and anti-blocking agent in the processing of polyethylene and other polymers. It reduces friction between polymer layers, improving the ease of processing and the quality of the final product .
Biology: In biological research, this compound has been studied for its role as a signaling molecule. It has been found to accumulate in the cerebrospinal fluid during sleep deprivation and is believed to induce sleep in animals .
Medicine: Oleamide has potential therapeutic applications due to its interaction with neurotransmitter systems. It has been investigated for its effects on mood and sleep disorders, as well as its potential role in cannabinoid-regulated depression .
Industry: In addition to its use in polymer processing, this compound is used as a lubricant, anti-static agent, and dispersant in various industrial applications. It is also used in the production of coatings, inks, and other fine chemicals .
Mechanism of Action
Target of Action
9-Octadecenamide, also known as oleamide , is a fatty acid amide derivative . These compounds are formed from a fatty acid and an amine . They are natural self-defense agents in plants and have been observed to be broadly bioactive against a variety of disease agents .
Mode of Action
The mechanism of action of this compound is understood to involve targeting protein synthesis and causing leakage of the intracellular components . This interaction with its targets leads to changes in the cellular environment and can disrupt the normal functioning of the cell .
Biochemical Pathways
It is known that fatty acid amides like this compound can have broad bioactivity, suggesting they may interact with multiple pathways
Result of Action
The molecular and cellular effects of this compound’s action are likely to be varied due to its broad bioactivity . Its ability to disrupt protein synthesis and cause leakage of intracellular components can lead to cell death . This makes this compound potentially useful in a variety of therapeutic applications.
Safety and Hazards
Biochemical Analysis
Biochemical Properties
9-Octadecenamide plays a significant role in biochemical reactions, particularly in the central nervous system. It interacts with several enzymes and proteins, including fatty acid amide hydrolase (FAAH), which is responsible for its rapid metabolism. The inhibition of FAAH by this compound leads to increased concentrations of other fatty acid amides, such as anandamide, which can influence various physiological processes .
Additionally, this compound has been shown to interact with cannabinoid receptors, particularly the CB1 receptor, although its affinity is relatively low. This interaction suggests that this compound may modulate neurotransmitter systems and contribute to its sleep-inducing effects .
Cellular Effects
This compound exerts various effects on different cell types and cellular processes. In glial cells, it has been found to selectively inhibit gap junction-mediated communication without affecting calcium wave transmission . This selective inhibition may play a role in regulating intercellular communication in the central nervous system and influencing higher-order neuronal events such as sleep induction .
Furthermore, this compound has been observed to enhance the activity of choline acetyltransferase, an enzyme critical for the production of acetylcholine, a neurotransmitter involved in many aspects of brain function . This enhancement may contribute to its effects on mood and cognitive function.
Molecular Mechanism
The molecular mechanism of this compound involves several pathways. One key mechanism is its interaction with FAAH, leading to the inhibition of this enzyme and subsequent increase in anandamide levels . Anandamide is an endocannabinoid that binds to cannabinoid receptors and modulates various physiological processes, including pain, mood, and appetite.
Additionally, this compound has been found to interact with the CB1 receptor, albeit with low affinity . This interaction may contribute to its effects on sleep and mood regulation. The compound also influences the activity of other neurotransmitter systems, including serotonin and dopamine, further contributing to its physiological effects.
Temporal Effects in Laboratory Settings
In laboratory settings, the effects of this compound have been studied over time to understand its stability, degradation, and long-term effects on cellular function. Studies have shown that this compound is rapidly metabolized by FAAH, leading to a relatively short duration of action . Its effects on cellular communication and neurotransmitter systems can have lasting impacts on physiological processes.
Long-term studies have indicated that chronic administration of this compound can lead to adaptive changes in the central nervous system, including alterations in receptor sensitivity and neurotransmitter levels . These changes may contribute to its therapeutic potential for mood and sleep disorders.
Dosage Effects in Animal Models
The effects of this compound vary with different dosages in animal models. At lower doses, it has been shown to induce sleep and reduce anxiety-like behaviors . At higher doses, it can lead to adverse effects, including hypothermia and motor impairment . These dose-dependent effects highlight the importance of careful dosage optimization for therapeutic applications.
Metabolic Pathways
This compound is involved in several metabolic pathways, primarily through its interaction with FAAH. The inhibition of FAAH by this compound leads to increased levels of other fatty acid amides, such as anandamide . This interaction can influence metabolic flux and alter the levels of various metabolites involved in physiological processes.
Additionally, this compound has been shown to enhance the activity of enzymes involved in neurotransmitter synthesis, such as choline acetyltransferase . This enhancement can impact the production and release of neurotransmitters, further contributing to its physiological effects.
Transport and Distribution
Within cells and tissues, this compound is transported and distributed through various mechanisms. It interacts with specific transporters and binding proteins that facilitate its movement across cell membranes and its accumulation in specific tissues . The compound’s lipophilic nature allows it to readily cross the blood-brain barrier, contributing to its effects on the central nervous system.
Subcellular Localization
The subcellular localization of this compound is influenced by its interactions with specific targeting signals and post-translational modifications. It has been found to localize in various cellular compartments, including the endoplasmic reticulum and mitochondria . These localizations can impact its activity and function, particularly in the context of neurotransmitter synthesis and cellular communication.
Preparation Methods
Synthetic Routes and Reaction Conditions: 9-Octadecenamide can be synthesized through the reaction of oleic acid with ammonia in the presence of a catalyst. Common catalysts include boric acid, activated alumina, and silica gel. The reaction proceeds through the formation of an ammonium salt intermediate, which then dehydrates to form the amide. The reaction typically occurs at temperatures between 170-200°C and pressures of 0.3-0.7 MPa over 10-12 hours .
Industrial Production Methods: In industrial settings, this compound is produced by reacting oleic acid with anhydrous ammonia in the presence of a catalyst. The reaction mixture is heated to remove water and unreacted ammonia, and the product is purified through recrystallization and filtration. The use of plant-derived oleic acid is preferred over animal-derived sources due to lower odor and better stability .
Chemical Reactions Analysis
Types of Reactions:
Hydrolysis: In the presence of acids, bases, or enzymes, 9-octadecenamide can undergo hydrolysis to yield oleic acid and ammonia.
Dehydration: When heated with strong dehydrating agents like phosphorus pentoxide, it forms nitriles.
Addition Reactions: It can react with formaldehyde or ethylene oxide to form various fine chemical products.
Common Reagents and Conditions:
Hydrolysis: Acidic or basic conditions, or enzymatic catalysis.
Dehydration: Strong dehydrating agents like phosphorus pentoxide.
Addition Reactions: Formaldehyde, ethylene oxide.
Major Products:
Hydrolysis: Oleic acid and ammonia.
Dehydration: Nitriles.
Addition Reactions: Various fine chemical products.
Comparison with Similar Compounds
- Erucamide (Z)-docos-13-enamide
- Stearamide (octadecanamide)
Comparison: While erucamide and stearamide are also fatty acid amides, 9-octadecenamide is unique in its bioactivity and its role as a signaling molecule. Erucamide is primarily used as a slip agent in polymer processing, while stearamide is used as a lubricant and anti-static agent. This compound’s ability to interact with neurotransmitter systems and its potential therapeutic applications set it apart from these other compounds .
Properties
IUPAC Name |
octadec-9-enamide | |
---|---|---|
Source | PubChem | |
URL | https://pubchem.ncbi.nlm.nih.gov | |
Description | Data deposited in or computed by PubChem | |
InChI |
InChI=1S/C18H35NO/c1-2-3-4-5-6-7-8-9-10-11-12-13-14-15-16-17-18(19)20/h9-10H,2-8,11-17H2,1H3,(H2,19,20) | |
Source | PubChem | |
URL | https://pubchem.ncbi.nlm.nih.gov | |
Description | Data deposited in or computed by PubChem | |
InChI Key |
FATBGEAMYMYZAF-UHFFFAOYSA-N | |
Source | PubChem | |
URL | https://pubchem.ncbi.nlm.nih.gov | |
Description | Data deposited in or computed by PubChem | |
Canonical SMILES |
CCCCCCCCC=CCCCCCCCC(=O)N | |
Source | PubChem | |
URL | https://pubchem.ncbi.nlm.nih.gov | |
Description | Data deposited in or computed by PubChem | |
Molecular Formula |
C18H35NO | |
Source | PubChem | |
URL | https://pubchem.ncbi.nlm.nih.gov | |
Description | Data deposited in or computed by PubChem | |
DSSTOX Substance ID |
DTXSID60859314 | |
Record name | Octadec-9-enamide | |
Source | EPA DSSTox | |
URL | https://comptox.epa.gov/dashboard/DTXSID60859314 | |
Description | DSSTox provides a high quality public chemistry resource for supporting improved predictive toxicology. | |
Molecular Weight |
281.5 g/mol | |
Source | PubChem | |
URL | https://pubchem.ncbi.nlm.nih.gov | |
Description | Data deposited in or computed by PubChem | |
CAS No. |
3322-62-1 | |
Record name | 9-Octadecenamide | |
Source | ChemIDplus | |
URL | https://pubchem.ncbi.nlm.nih.gov/substance/?source=chemidplus&sourceid=0003322621 | |
Description | ChemIDplus is a free, web search system that provides access to the structure and nomenclature authority files used for the identification of chemical substances cited in National Library of Medicine (NLM) databases, including the TOXNET system. | |
Record name | Octadec-9-enamide | |
Source | EPA DSSTox | |
URL | https://comptox.epa.gov/dashboard/DTXSID60859314 | |
Description | DSSTox provides a high quality public chemistry resource for supporting improved predictive toxicology. | |
Retrosynthesis Analysis
AI-Powered Synthesis Planning: Our tool employs the Template_relevance Pistachio, Template_relevance Bkms_metabolic, Template_relevance Pistachio_ringbreaker, Template_relevance Reaxys, Template_relevance Reaxys_biocatalysis model, leveraging a vast database of chemical reactions to predict feasible synthetic routes.
One-Step Synthesis Focus: Specifically designed for one-step synthesis, it provides concise and direct routes for your target compounds, streamlining the synthesis process.
Accurate Predictions: Utilizing the extensive PISTACHIO, BKMS_METABOLIC, PISTACHIO_RINGBREAKER, REAXYS, REAXYS_BIOCATALYSIS database, our tool offers high-accuracy predictions, reflecting the latest in chemical research and data.
Strategy Settings
Precursor scoring | Relevance Heuristic |
---|---|
Min. plausibility | 0.01 |
Model | Template_relevance |
Template Set | Pistachio/Bkms_metabolic/Pistachio_ringbreaker/Reaxys/Reaxys_biocatalysis |
Top-N result to add to graph | 6 |
Feasible Synthetic Routes
ANone: While 9-octadecenamide is not typically considered a catalyst, it plays a role in biological systems, particularly as a signaling molecule. Its functions are closely tied to its interactions with enzymes and receptors rather than direct catalytic activity.
A: Yes, computational methods have been employed to design and study hydrolysis-resistant analogs of this compound, aiming to develop novel orally active PPARα ligands. []
A: Research has focused on developing analogs of this compound with improved hydrolysis resistance. This approach aims to enhance the compound's stability and potentially its in vivo efficacy for therapeutic applications. []
ANone: While specific SHE regulations for this compound might vary, its use in research and potential applications should adhere to general laboratory safety practices and guidelines for handling chemicals.
ANone: Further research is required to comprehensively evaluate the long-term safety profile of this compound, particularly in the context of potential therapeutic applications.
A: Researchers have focused on developing hydrolysis-resistant analogs of this compound to improve its stability and potentially enhance its delivery to target tissues, especially for oral administration. []
A: Doublecortin (DCX) has emerged as a potential biomarker for monitoring the effects of this compound on hippocampal neurogenesis, particularly in the context of its sleep-enhancing properties. []
ANone: More research is needed to fully understand the fate and ecological effects of this compound in various environmental compartments.
ANone: this compound, being a lipophilic compound, exhibits limited solubility in aqueous solutions. This property can influence its bioavailability and necessitates the exploration of strategies to improve its solubility for therapeutic applications.
ANone: Validation of analytical methods, such as GC-MS, for this compound quantification typically involves assessing parameters such as accuracy, precision, specificity, linearity, range, limit of detection, and limit of quantification to ensure reliable and reproducible results.
ANone: If this compound were to be developed for pharmaceutical use, robust quality control and assurance measures would be essential throughout its development, manufacturing, and distribution to ensure its safety, efficacy, and consistency.
ANone: Currently, there is limited information available on the potential immunogenicity of this compound. Further studies are required to assess its potential to elicit immune responses.
A: Research indicates that this compound interacts with the fatty acid transporter CD36, influencing its uptake into intestinal epithelial cells. This interaction could potentially affect its absorption and bioavailability. []
ANone: Further research is necessary to determine if this compound has any direct effects on the expression or activity of drug-metabolizing enzymes.
A: As a naturally occurring lipid, this compound generally exhibits good biocompatibility. It is readily metabolized by enzymes present in biological systems, suggesting it is biodegradable. [, ]
ANone: Specific guidelines for handling and disposal of this compound should be followed, adhering to regulations for chemical waste management to minimize environmental impact.
ANone: Continued research on this compound would benefit from access to advanced analytical techniques such as GC-MS, LC-MS, and high-throughput screening platforms, as well as collaborations with experts in fields such as lipidomics, pharmacology, and neuroscience.
A: The discovery of this compound as an endogenous sleep-inducing lipid, its characterization as a PPARα agonist, and the development of hydrolysis-resistant analogs represent significant milestones in understanding its biological roles and therapeutic potential. [, , ]
Disclaimer and Information on In-Vitro Research Products
Please be aware that all articles and product information presented on BenchChem are intended solely for informational purposes. The products available for purchase on BenchChem are specifically designed for in-vitro studies, which are conducted outside of living organisms. In-vitro studies, derived from the Latin term "in glass," involve experiments performed in controlled laboratory settings using cells or tissues. It is important to note that these products are not categorized as medicines or drugs, and they have not received approval from the FDA for the prevention, treatment, or cure of any medical condition, ailment, or disease. We must emphasize that any form of bodily introduction of these products into humans or animals is strictly prohibited by law. It is essential to adhere to these guidelines to ensure compliance with legal and ethical standards in research and experimentation.