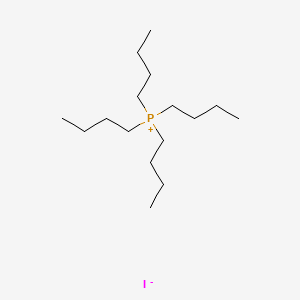
Tetrabutylphosphonium iodide
Overview
Description
Tetrabutylphosphonium iodide is a chemical compound with the molecular formula C16H36IP. It is a white crystalline solid known for its applications in various chemical processes. This compound is characterized by its phosphonium and iodide functional groups, making it a valuable reagent in both academic and industrial settings .
Mechanism of Action
Target of Action
Tetrabutylphosphonium iodide is primarily used as an interfacial additive in the field of perovskite solar cells . Its primary targets are the electron selective contact – mesoporous – TiO2 and the perovskite CH3NH3PbI3 active layer .
Mode of Action
This compound interacts with its targets by being applied as an additive at the interface between the electron selective contact – mesoporous – TiO2 and the perovskite CH3NH3PbI3 active layer . This interaction results in an improved device stability and a reduction of the hysteretic behavior, suggesting a possible passivation of trap states and/or mitigation of ionic migration phenomena .
Biochemical Pathways
The specific conditions of this compound interfacial modification result in an improved device stability, suggesting that it may affect the pathways related to the stability of these devices .
Pharmacokinetics
It is known that the compound is used as an additive in perovskite solar cells, suggesting that its distribution and metabolism are likely related to its role in these devices .
Result of Action
The application of this compound as an interfacial additive results in an improved device stability and a reduction of the hysteretic behavior in perovskite solar cells . This suggests that the compound’s action leads to a possible passivation of trap states and/or mitigation of ionic migration phenomena .
Action Environment
The action of this compound is influenced by the specific conditions of its interfacial modification .
Biochemical Analysis
Biochemical Properties
Tetrabutylphosphonium iodide plays a significant role in biochemical reactions due to its ability to interact with various enzymes, proteins, and other biomolecules. It has been observed to influence the physico-chemical properties of amino acid ionic liquids, leading to lower viscosities and higher decomposition temperatures . The compound interacts with enzymes such as phosphatases and oxidoreductases, affecting their activity and stability. These interactions are primarily driven by the ionic nature of this compound, which can alter the local environment of the enzymes, leading to changes in their conformation and activity.
Cellular Effects
This compound has been shown to impact various cellular processes, including cell signaling pathways, gene expression, and cellular metabolism. In studies involving mesoscopic perovskite solar cells, the compound was used as an interfacial additive, resulting in improved device stability and reduced hysteretic behavior . This suggests that this compound can influence cell signaling pathways by modulating the interactions between different cellular components. Additionally, the compound has been found to affect gene expression by altering the transcriptional activity of specific genes involved in cellular metabolism and stress responses.
Molecular Mechanism
Temporal Effects in Laboratory Settings
In laboratory settings, the effects of this compound have been observed to change over time. The compound exhibits high stability and low degradation rates, making it suitable for long-term studies . Prolonged exposure to this compound can lead to gradual changes in cellular function, including alterations in metabolic activity and gene expression. These long-term effects are likely due to the accumulation of the compound within cells and tissues, which can affect the overall cellular environment and the activity of various biomolecules.
Dosage Effects in Animal Models
The effects of this compound in animal models vary with different dosages. At low doses, the compound has been found to enhance cellular function and improve metabolic activity. At high doses, this compound can exhibit toxic effects, including cellular damage and impaired organ function . These adverse effects are likely due to the accumulation of the compound within tissues, leading to disruptions in cellular homeostasis and the activation of stress response pathways.
Metabolic Pathways
This compound is involved in various metabolic pathways, including those related to the synthesis and degradation of biomolecules. The compound interacts with enzymes such as oxidoreductases and transferases, influencing their activity and the overall metabolic flux . These interactions can lead to changes in metabolite levels and the regulation of metabolic pathways, affecting cellular function and homeostasis.
Transport and Distribution
Within cells and tissues, this compound is transported and distributed through interactions with various transporters and binding proteins. The compound’s ionic nature allows it to be readily taken up by cells and distributed throughout different cellular compartments . This distribution is influenced by the presence of specific transporters and binding proteins, which can facilitate the movement of this compound across cellular membranes and its accumulation in specific tissues.
Subcellular Localization
The subcellular localization of this compound is determined by its interactions with targeting signals and post-translational modifications. The compound can be directed to specific cellular compartments, such as the nucleus, mitochondria, or endoplasmic reticulum, where it can exert its effects on cellular function . These localization signals are essential for the proper functioning of this compound, as they ensure that the compound reaches its target sites within the cell and interacts with the appropriate biomolecules.
Preparation Methods
Synthetic Routes and Reaction Conditions: Tetrabutylphosphonium iodide can be synthesized through the reaction of 1-iodobutane with tributylphosphine. The reaction is typically carried out under an inert atmosphere, such as argon, to prevent oxidation. The mixture is stirred at room temperature and then heated to 100°C for 20 hours to ensure complete reaction .
Industrial Production Methods: In industrial settings, the synthesis of this compound follows similar principles but on a larger scale. The reaction conditions are optimized to maximize yield and purity, often involving continuous stirring and precise temperature control. The product is then purified through recrystallization or other suitable methods to obtain the desired quality .
Chemical Reactions Analysis
Types of Reactions: Tetrabutylphosphonium iodide undergoes various chemical reactions, including:
Substitution Reactions: It can participate in nucleophilic substitution reactions where the iodide ion is replaced by other nucleophiles.
Oxidation and Reduction Reactions: The phosphonium ion can undergo redox reactions, although these are less common compared to substitution reactions.
Common Reagents and Conditions:
Nucleophiles: Common nucleophiles used in substitution reactions include halides, cyanides, and alkoxides.
Oxidizing Agents: For oxidation reactions, agents like hydrogen peroxide or potassium permanganate may be used.
Reducing Agents: Reducing agents such as lithium aluminum hydride can be employed for reduction reactions.
Major Products: The major products formed from these reactions depend on the specific reagents and conditions used. For example, substitution reactions with halides can yield various phosphonium halides, while oxidation reactions may produce phosphine oxides .
Scientific Research Applications
Tetrabutylphosphonium iodide has a wide range of applications in scientific research:
Biology: In biological research, it can be used to modify biomolecules or as a reagent in various biochemical assays.
Comparison with Similar Compounds
- Tetrabutylphosphonium Bromide
- Tetrabutylphosphonium Chloride
- Tetrabutylphosphonium Fluoride
Comparison: Tetrabutylphosphonium iodide is unique due to its iodide ion, which imparts distinct reactivity compared to its bromide, chloride, and fluoride counterparts. The iodide ion is a better leaving group in substitution reactions, making this compound more reactive in such processes. Additionally, the iodide ion’s larger size and lower electronegativity compared to bromide, chloride, and fluoride ions can influence the compound’s solubility and interaction with other molecules .
Properties
IUPAC Name |
tetrabutylphosphanium;iodide | |
---|---|---|
Source | PubChem | |
URL | https://pubchem.ncbi.nlm.nih.gov | |
Description | Data deposited in or computed by PubChem | |
InChI |
InChI=1S/C16H36P.HI/c1-5-9-13-17(14-10-6-2,15-11-7-3)16-12-8-4;/h5-16H2,1-4H3;1H/q+1;/p-1 | |
Source | PubChem | |
URL | https://pubchem.ncbi.nlm.nih.gov | |
Description | Data deposited in or computed by PubChem | |
InChI Key |
CCIYPTIBRAUPLQ-UHFFFAOYSA-M | |
Source | PubChem | |
URL | https://pubchem.ncbi.nlm.nih.gov | |
Description | Data deposited in or computed by PubChem | |
Canonical SMILES |
CCCC[P+](CCCC)(CCCC)CCCC.[I-] | |
Source | PubChem | |
URL | https://pubchem.ncbi.nlm.nih.gov | |
Description | Data deposited in or computed by PubChem | |
Molecular Formula |
C16H36IP | |
Source | PubChem | |
URL | https://pubchem.ncbi.nlm.nih.gov | |
Description | Data deposited in or computed by PubChem | |
Related CAS |
3115-68-2 (Parent) | |
Record name | Phosphonium, tetrabutyl-, iodide | |
Source | ChemIDplus | |
URL | https://pubchem.ncbi.nlm.nih.gov/substance/?source=chemidplus&sourceid=0003115660 | |
Description | ChemIDplus is a free, web search system that provides access to the structure and nomenclature authority files used for the identification of chemical substances cited in National Library of Medicine (NLM) databases, including the TOXNET system. | |
DSSTOX Substance ID |
DTXSID20953241 | |
Record name | Tetrabutylphosphanium iodide | |
Source | EPA DSSTox | |
URL | https://comptox.epa.gov/dashboard/DTXSID20953241 | |
Description | DSSTox provides a high quality public chemistry resource for supporting improved predictive toxicology. | |
Molecular Weight |
386.33 g/mol | |
Source | PubChem | |
URL | https://pubchem.ncbi.nlm.nih.gov | |
Description | Data deposited in or computed by PubChem | |
CAS No. |
3115-66-0 | |
Record name | Phosphonium, tetrabutyl-, iodide (1:1) | |
Source | CAS Common Chemistry | |
URL | https://commonchemistry.cas.org/detail?cas_rn=3115-66-0 | |
Description | CAS Common Chemistry is an open community resource for accessing chemical information. Nearly 500,000 chemical substances from CAS REGISTRY cover areas of community interest, including common and frequently regulated chemicals, and those relevant to high school and undergraduate chemistry classes. This chemical information, curated by our expert scientists, is provided in alignment with our mission as a division of the American Chemical Society. | |
Explanation | The data from CAS Common Chemistry is provided under a CC-BY-NC 4.0 license, unless otherwise stated. | |
Record name | Phosphonium, tetrabutyl-, iodide | |
Source | ChemIDplus | |
URL | https://pubchem.ncbi.nlm.nih.gov/substance/?source=chemidplus&sourceid=0003115660 | |
Description | ChemIDplus is a free, web search system that provides access to the structure and nomenclature authority files used for the identification of chemical substances cited in National Library of Medicine (NLM) databases, including the TOXNET system. | |
Record name | Tetrabutylphosphonium iodide | |
Source | DTP/NCI | |
URL | https://dtp.cancer.gov/dtpstandard/servlet/dwindex?searchtype=NSC&outputformat=html&searchlist=104836 | |
Description | The NCI Development Therapeutics Program (DTP) provides services and resources to the academic and private-sector research communities worldwide to facilitate the discovery and development of new cancer therapeutic agents. | |
Explanation | Unless otherwise indicated, all text within NCI products is free of copyright and may be reused without our permission. Credit the National Cancer Institute as the source. | |
Record name | Tetrabutylphosphanium iodide | |
Source | EPA DSSTox | |
URL | https://comptox.epa.gov/dashboard/DTXSID20953241 | |
Description | DSSTox provides a high quality public chemistry resource for supporting improved predictive toxicology. | |
Retrosynthesis Analysis
AI-Powered Synthesis Planning: Our tool employs the Template_relevance Pistachio, Template_relevance Bkms_metabolic, Template_relevance Pistachio_ringbreaker, Template_relevance Reaxys, Template_relevance Reaxys_biocatalysis model, leveraging a vast database of chemical reactions to predict feasible synthetic routes.
One-Step Synthesis Focus: Specifically designed for one-step synthesis, it provides concise and direct routes for your target compounds, streamlining the synthesis process.
Accurate Predictions: Utilizing the extensive PISTACHIO, BKMS_METABOLIC, PISTACHIO_RINGBREAKER, REAXYS, REAXYS_BIOCATALYSIS database, our tool offers high-accuracy predictions, reflecting the latest in chemical research and data.
Strategy Settings
Precursor scoring | Relevance Heuristic |
---|---|
Min. plausibility | 0.01 |
Model | Template_relevance |
Template Set | Pistachio/Bkms_metabolic/Pistachio_ringbreaker/Reaxys/Reaxys_biocatalysis |
Top-N result to add to graph | 6 |
Feasible Synthetic Routes
Q1: How does Tetrabutylphosphonium iodide improve the performance of perovskite solar cells?
A: While the provided abstracts don't delve into specific performance improvements, they highlight that this compound is explored as an interfacial additive between Titanium dioxide (TiO2) and Methylammonium lead iodide (CH3NH3PbI3) in mesoscopic perovskite solar cells [, ]. This suggests its role is likely at the interface of these two materials, potentially influencing charge transport, reducing recombination losses, or improving the stability of the perovskite layer. Further research is needed to elucidate the precise mechanisms of improvement.
Disclaimer and Information on In-Vitro Research Products
Please be aware that all articles and product information presented on BenchChem are intended solely for informational purposes. The products available for purchase on BenchChem are specifically designed for in-vitro studies, which are conducted outside of living organisms. In-vitro studies, derived from the Latin term "in glass," involve experiments performed in controlled laboratory settings using cells or tissues. It is important to note that these products are not categorized as medicines or drugs, and they have not received approval from the FDA for the prevention, treatment, or cure of any medical condition, ailment, or disease. We must emphasize that any form of bodily introduction of these products into humans or animals is strictly prohibited by law. It is essential to adhere to these guidelines to ensure compliance with legal and ethical standards in research and experimentation.