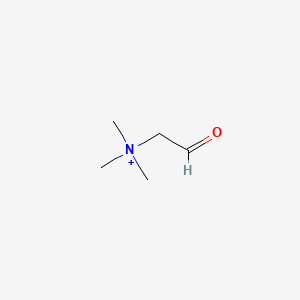
Betaine aldehyde
Overview
Description
Betaine aldehyde, also known as glycine this compound, is an intermediate in the metabolism of glycine, serine, and threonine. It is a short-chain aldehyde and a quaternary ammonium compound. This compound is a substrate for choline dehydrogenase (mitochondrial) and is involved in the biosynthesis of glycine betaine, an important osmoprotectant .
Scientific Research Applications
Betaine aldehyde has several scientific research applications, including:
Preparation Methods
Synthetic Routes and Reaction Conditions
Betaine aldehyde can be synthesized through the oxidation of choline. The process involves two key enzymes: choline dehydrogenase and this compound dehydrogenase. Choline is first oxidized to this compound by choline dehydrogenase, and then this compound is further oxidized to glycine betaine by this compound dehydrogenase .
Industrial Production Methods
Industrial production of this compound typically involves the extraction from natural sources such as sugar beets (Beta vulgaris). The extraction process includes accelerated solvent extraction coupled with solid-phase extraction to isolate and purify betaine from plant materials .
Chemical Reactions Analysis
Types of Reactions
Betaine aldehyde undergoes several types of chemical reactions, including:
Oxidation: This compound is oxidized to glycine betaine by this compound dehydrogenase.
Reduction: this compound can be reduced to choline under specific conditions.
Substitution: this compound can participate in nucleophilic substitution reactions due to the presence of the aldehyde group.
Common Reagents and Conditions
Oxidation: Common reagents include choline dehydrogenase and this compound dehydrogenase.
Reduction: Reducing agents such as sodium borohydride can be used.
Substitution: Nucleophiles such as amines can react with this compound under mild conditions.
Major Products Formed
Oxidation: Glycine betaine is the major product formed from the oxidation of this compound.
Reduction: Choline is formed from the reduction of this compound.
Substitution: Various substituted derivatives of this compound can be formed depending on the nucleophile used.
Mechanism of Action
Betaine aldehyde exerts its effects primarily through its conversion to glycine betaine. Glycine betaine acts as an osmoprotectant, stabilizing cellular structures and protecting cells from osmotic stress. The conversion involves the enzyme this compound dehydrogenase, which catalyzes the oxidation of this compound to glycine betaine . This process is crucial for maintaining cellular homeostasis under stress conditions.
Comparison with Similar Compounds
Similar Compounds
Glycine Betaine: A direct product of betaine aldehyde oxidation, known for its osmoprotective properties.
Choline: A precursor to this compound, involved in various metabolic pathways.
Trimethylglycine: Another name for glycine betaine, highlighting its methylated structure.
Uniqueness
This compound is unique due to its role as an intermediate in the biosynthesis of glycine betaine. Its ability to undergo oxidation and reduction reactions makes it a versatile compound in metabolic pathways. Additionally, its involvement in osmoprotection and stress response mechanisms sets it apart from other similar compounds .
Properties
CAS No. |
7418-61-3 |
---|---|
Molecular Formula |
C5H12NO+ |
Molecular Weight |
102.15 g/mol |
IUPAC Name |
trimethyl(2-oxoethyl)azanium |
InChI |
InChI=1S/C5H12NO/c1-6(2,3)4-5-7/h5H,4H2,1-3H3/q+1 |
InChI Key |
SXKNCCSPZDCRFD-UHFFFAOYSA-N |
SMILES |
C[N+](C)(C)CC=O |
Canonical SMILES |
C[N+](C)(C)CC=O |
7418-61-3 | |
physical_description |
Solid |
Synonyms |
etaine aldehyde glycine betaine aldehyde |
Origin of Product |
United States |
Synthesis routes and methods
Procedure details
Retrosynthesis Analysis
AI-Powered Synthesis Planning: Our tool employs the Template_relevance Pistachio, Template_relevance Bkms_metabolic, Template_relevance Pistachio_ringbreaker, Template_relevance Reaxys, Template_relevance Reaxys_biocatalysis model, leveraging a vast database of chemical reactions to predict feasible synthetic routes.
One-Step Synthesis Focus: Specifically designed for one-step synthesis, it provides concise and direct routes for your target compounds, streamlining the synthesis process.
Accurate Predictions: Utilizing the extensive PISTACHIO, BKMS_METABOLIC, PISTACHIO_RINGBREAKER, REAXYS, REAXYS_BIOCATALYSIS database, our tool offers high-accuracy predictions, reflecting the latest in chemical research and data.
Strategy Settings
Precursor scoring | Relevance Heuristic |
---|---|
Min. plausibility | 0.01 |
Model | Template_relevance |
Template Set | Pistachio/Bkms_metabolic/Pistachio_ringbreaker/Reaxys/Reaxys_biocatalysis |
Top-N result to add to graph | 6 |
Feasible Synthetic Routes
Disclaimer and Information on In-Vitro Research Products
Please be aware that all articles and product information presented on BenchChem are intended solely for informational purposes. The products available for purchase on BenchChem are specifically designed for in-vitro studies, which are conducted outside of living organisms. In-vitro studies, derived from the Latin term "in glass," involve experiments performed in controlled laboratory settings using cells or tissues. It is important to note that these products are not categorized as medicines or drugs, and they have not received approval from the FDA for the prevention, treatment, or cure of any medical condition, ailment, or disease. We must emphasize that any form of bodily introduction of these products into humans or animals is strictly prohibited by law. It is essential to adhere to these guidelines to ensure compliance with legal and ethical standards in research and experimentation.