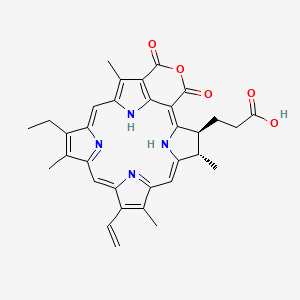
Purpurin 18
Overview
Description
Purpurin 18 is a chlorophyll metabolite and a potent photosensitizer widely used in photodynamic therapy (PDT) for cancer treatment. It is derived from natural sources such as green plants, including seaweed and spinach leaves . This compound is known for its ability to generate reactive oxygen species upon light activation, making it effective in selectively destroying tumor cells .
Mechanism of Action
Target of Action
Purpurin 18 (P18) is a potent natural product and a chlorophyll metabolite . It is primarily used as a photosensitizer in photodynamic therapy (PDT) for cancer treatment . The primary targets of P18 are the lysosomes, mitochondria, Golgi apparatus, and endoplasmic reticulum within the cancer cells .
Mode of Action
P18 interacts with its targets by distributing itself across the lysosomes, mitochondria, Golgi apparatus, and endoplasmic reticulum . Upon light irradiation, P18 generates reactive oxygen species (ROS) that destroy the mitochondrial membrane potential (MMP), leading to cell apoptosis .
Biochemical Pathways
The biochemical pathways affected by P18 primarily involve the generation of ROS. The ROS produced by P18 upon light irradiation disrupts the MMP, which is a crucial component of the cell’s energy production pathway . This disruption leads to cell apoptosis, effectively killing the cancer cells .
Pharmacokinetics
P18 is hydrophobic, which can lead to easy coalescence and poor bioavailability . To improve its bioavailability, P18 can be loaded into solid lipid nanoparticles (SLNs) . These P18-loaded SLNs have suitable properties for an enhanced permeability and retention effect, making them an effective passive targeting anti-cancer strategy .
Result of Action
The result of P18’s action is the induction of apoptosis in cancer cells . In vitro studies have shown that P18 can significantly inhibit the growth of triple negative breast cancer cells . In vivo studies have also demonstrated that P18 can significantly inhibit tumor growth without influencing weight and major organ function .
Action Environment
The action of P18 is influenced by environmental factors such as pH. P18 is stable at acidic pH (6.5), and its photodynamic efficacy improves when it is entrapped in SLNs . Furthermore, P18 can remain in the tumor for more than 4 days by direct intra-tumoral injection, allowing for effective PDT treatment .
Biochemical Analysis
Biochemical Properties
Purpurin 18 plays a crucial role in biochemical reactions, particularly in the context of photodynamic therapy. As a photosensitizer, this compound interacts with light to produce reactive oxygen species (ROS), which can induce cell death in targeted tissues. The compound interacts with various biomolecules, including enzymes and proteins, to exert its effects. For instance, this compound has been shown to interact with enzymes involved in the generation of ROS, such as singlet oxygen . Additionally, it can bind to proteins and other biomolecules, facilitating its transport and distribution within cells .
Cellular Effects
This compound influences various cellular processes, including cell signaling pathways, gene expression, and cellular metabolism. In cancer cells, this compound has been observed to induce apoptosis through the generation of ROS, which disrupts mitochondrial membrane potential and activates apoptotic pathways . The compound also affects cell signaling pathways by modulating the activity of key signaling molecules, leading to changes in gene expression and cellular metabolism . These effects contribute to the selective destruction of cancer cells while sparing healthy tissues.
Molecular Mechanism
At the molecular level, this compound exerts its effects through several mechanisms. The compound binds to cellular membranes and organelles, where it generates ROS upon light activation . This ROS production leads to oxidative damage to cellular components, including lipids, proteins, and DNA, ultimately resulting in cell death. This compound also interacts with enzymes involved in ROS generation, enhancing its photodynamic efficacy . Additionally, the compound can modulate gene expression by affecting transcription factors and other regulatory proteins .
Temporal Effects in Laboratory Settings
In laboratory settings, the effects of this compound can vary over time. The compound’s stability and degradation are critical factors that influence its efficacy. Studies have shown that this compound remains stable under specific conditions, allowing for sustained ROS production and prolonged photodynamic effects . Over extended periods, the compound may degrade, leading to reduced efficacy. Long-term studies have demonstrated that this compound can induce lasting changes in cellular function, including alterations in gene expression and metabolic activity .
Dosage Effects in Animal Models
The effects of this compound vary with different dosages in animal models. At low doses, the compound exhibits minimal toxicity and effectively induces apoptosis in cancer cells . At higher doses, this compound can cause adverse effects, including oxidative damage to healthy tissues and systemic toxicity . Threshold effects have been observed, where a specific dosage range maximizes the compound’s therapeutic efficacy while minimizing adverse effects .
Metabolic Pathways
This compound is involved in several metabolic pathways, primarily related to its role as a photosensitizer. The compound interacts with enzymes and cofactors involved in ROS generation, such as singlet oxygen . These interactions enhance the compound’s photodynamic activity and contribute to its therapeutic effects. Additionally, this compound can affect metabolic flux and metabolite levels by modulating the activity of key metabolic enzymes .
Transport and Distribution
Within cells and tissues, this compound is transported and distributed through interactions with transporters and binding proteins . The compound’s hydrophobic nature allows it to associate with cellular membranes, facilitating its uptake and distribution. Once inside the cell, this compound can accumulate in specific organelles, such as mitochondria and lysosomes, where it exerts its photodynamic effects . These interactions are crucial for the compound’s localization and therapeutic efficacy.
Subcellular Localization
This compound exhibits specific subcellular localization, which is vital for its activity and function. The compound has been observed to localize in organelles such as mitochondria, lysosomes, Golgi apparatus, and endoplasmic reticulum . This localization is essential for its photodynamic efficacy, as it determines the primary sites of ROS generation and oxidative damage. Targeting signals and post-translational modifications may direct this compound to these specific compartments, enhancing its therapeutic potential .
Preparation Methods
Synthetic Routes and Reaction Conditions: Purpurin 18 can be synthesized from chlorophyll derivatives through a series of chemical reactions. One common method involves the extraction of chlorophyll derivatives from plant raw materials, followed by their oxidation in an alcoholic alkaline medium using air oxygen. The resulting unstable chlorin is then converted to this compound, which is purified on silica gel and crystallized . Another method involves the use of intact Chlorella cells to extract chlorophyll α, which is then processed through methyl pheophorbide or ethyl pheophorbide to produce this compound .
Industrial Production Methods: Industrial production of this compound typically involves large-scale extraction from plant sources, followed by chemical processing to obtain the final product. The use of solid lipid nanoparticles (SLNs) has been explored to improve the bioavailability and stability of this compound for industrial applications .
Chemical Reactions Analysis
Types of Reactions: Purpurin 18 undergoes various chemical reactions, including electrophilic addition, 1,3-polar cycloaddition on the vinyl group at the 3-position, and electrophilic substitution at the 20-meso-position . These reactions are essential for modifying the compound to enhance its properties for specific applications.
Common Reagents and Conditions: Common reagents used in the chemical reactions of this compound include dichloromethane (CH₂Cl₂), hydrochloric acid (HCl), sodium bicarbonate (NaHCO₃), and sodium sulfate (Na₂SO₄) . The reactions are typically carried out under controlled conditions to ensure the desired product is obtained.
Major Products: The major products formed from the chemical reactions of this compound include various derivatives that exhibit improved solubility, stability, and photodynamic efficacy. These derivatives are often used in photodynamic therapy for cancer treatment .
Scientific Research Applications
Purpurin 18 has a wide range of scientific research applications, particularly in the fields of chemistry, biology, medicine, and industry. Some of the key applications include:
Photodynamic Therapy (PDT): this compound is extensively used as a photosensitizer in PDT for cancer treatment.
Tumor Imaging and Diagnosis: this compound and its derivatives are used in tumor imaging and diagnosis due to their ability to target and accumulate in tumor tissues.
Drug Delivery Systems: this compound-loaded solid lipid nanoparticles (SLNs) have been developed to improve the bioavailability and stability of the compound for anticancer therapy.
Research on Reactive Oxygen Species: this compound is used in research to study the generation and effects of reactive oxygen species in biological systems.
Comparison with Similar Compounds
Purpurin 18 is unique among photosensitizers due to its natural origin and potent photodynamic efficacy. Similar compounds include other chlorophyll metabolites and synthetic photosensitizers used in PDT. Some of the similar compounds are:
Chlorin e6: Another chlorophyll-derived photosensitizer used in PDT.
Hematoporphyrin Derivative (HPD): A synthetic photosensitizer used in early PDT applications.
Photofrin: A commercially available photosensitizer used in clinical PDT.
This compound stands out due to its higher hydrophilicity and improved solubility when modified with polyethylene glycol (PEG) linkers, enhancing its photodynamic efficacy .
Properties
IUPAC Name |
3-[(22S,23S)-17-ethenyl-12-ethyl-13,18,22,27-tetramethyl-3,5-dioxo-4-oxa-8,24,25,26-tetrazahexacyclo[19.2.1.16,9.111,14.116,19.02,7]heptacosa-1,6,9(27),10,12,14(26),15,17,19(25),20-decaen-23-yl]propanoic acid | |
---|---|---|
Source | PubChem | |
URL | https://pubchem.ncbi.nlm.nih.gov | |
Description | Data deposited in or computed by PubChem | |
InChI |
InChI=1S/C33H32N4O5/c1-7-18-14(3)21-11-23-16(5)20(9-10-27(38)39)30(36-23)29-31-28(32(40)42-33(29)41)17(6)24(37-31)13-26-19(8-2)15(4)22(35-26)12-25(18)34-21/h7,11-13,16,20,36-37H,1,8-10H2,2-6H3,(H,38,39)/t16-,20-/m0/s1 | |
Source | PubChem | |
URL | https://pubchem.ncbi.nlm.nih.gov | |
Description | Data deposited in or computed by PubChem | |
InChI Key |
KUFHJWJISCAHNP-JXFKEZNVSA-N | |
Source | PubChem | |
URL | https://pubchem.ncbi.nlm.nih.gov | |
Description | Data deposited in or computed by PubChem | |
Canonical SMILES |
CCC1=C(C2=NC1=CC3=C(C4=C(N3)C(=C5C(C(C(=CC6=NC(=C2)C(=C6C)C=C)N5)C)CCC(=O)O)C(=O)OC4=O)C)C | |
Source | PubChem | |
URL | https://pubchem.ncbi.nlm.nih.gov | |
Description | Data deposited in or computed by PubChem | |
Isomeric SMILES |
CCC1=C(C2=NC1=CC3=C(C4=C(N3)C(=C5[C@H]([C@@H](C(=CC6=NC(=C2)C(=C6C)C=C)N5)C)CCC(=O)O)C(=O)OC4=O)C)C | |
Source | PubChem | |
URL | https://pubchem.ncbi.nlm.nih.gov | |
Description | Data deposited in or computed by PubChem | |
Molecular Formula |
C33H32N4O5 | |
Source | PubChem | |
URL | https://pubchem.ncbi.nlm.nih.gov | |
Description | Data deposited in or computed by PubChem | |
Molecular Weight |
564.6 g/mol | |
Source | PubChem | |
URL | https://pubchem.ncbi.nlm.nih.gov | |
Description | Data deposited in or computed by PubChem | |
CAS No. |
25465-77-4 | |
Record name | Purpurin 18 | |
Source | ChemIDplus | |
URL | https://pubchem.ncbi.nlm.nih.gov/substance/?source=chemidplus&sourceid=0025465774 | |
Description | ChemIDplus is a free, web search system that provides access to the structure and nomenclature authority files used for the identification of chemical substances cited in National Library of Medicine (NLM) databases, including the TOXNET system. | |
Retrosynthesis Analysis
AI-Powered Synthesis Planning: Our tool employs the Template_relevance Pistachio, Template_relevance Bkms_metabolic, Template_relevance Pistachio_ringbreaker, Template_relevance Reaxys, Template_relevance Reaxys_biocatalysis model, leveraging a vast database of chemical reactions to predict feasible synthetic routes.
One-Step Synthesis Focus: Specifically designed for one-step synthesis, it provides concise and direct routes for your target compounds, streamlining the synthesis process.
Accurate Predictions: Utilizing the extensive PISTACHIO, BKMS_METABOLIC, PISTACHIO_RINGBREAKER, REAXYS, REAXYS_BIOCATALYSIS database, our tool offers high-accuracy predictions, reflecting the latest in chemical research and data.
Strategy Settings
Precursor scoring | Relevance Heuristic |
---|---|
Min. plausibility | 0.01 |
Model | Template_relevance |
Template Set | Pistachio/Bkms_metabolic/Pistachio_ringbreaker/Reaxys/Reaxys_biocatalysis |
Top-N result to add to graph | 6 |
Feasible Synthetic Routes
A: Purpurin 18 acts as a photosensitizer in photodynamic therapy (PDT). [, , , ] Upon irradiation with light of a specific wavelength, it gets excited and transfers energy to surrounding oxygen molecules, generating reactive oxygen species (ROS) like singlet oxygen. [, , ] This leads to oxidative stress and damage to cellular components, particularly mitochondria and the endoplasmic reticulum, ultimately inducing cell death via apoptosis. [, , ]
ANone:
- Molecular Formula: C34H34N4O6 [, , ]
- Molecular Weight: 594.65 g/mol [, ]
- Spectroscopic Data:
- UV-Vis Absorption: Maximum absorption in the red region, ranging from 695 nm to 718 nm depending on the solvent and modifications. [, , , ]
- Fluorescence: Emits in the red to near-infrared region, making it suitable for fluorescence imaging. [, , , ]
- NMR: Detailed structural information can be obtained from 1H NMR and 13C NMR spectroscopy. [, , , ]
- Mass Spectrometry: FAB-MS is commonly used to confirm the molecular weight and identify fragments. [, ]
ANone:
- Stability:
- Compatibility:
A:
- QSAR models: Researchers have developed Quantitative Structure-Activity Relationship (QSAR) models to predict the photodynamic activity of this compound derivatives based on their structural features. [, ]
- Lipophilicity calculations: Computational tools like the PALLAS program have been used to calculate the log P and log D values of this compound derivatives, which are important for understanding their biodistribution and PDT efficacy. []
A:
- PEGylation: Increases water solubility and enhances cellular uptake, leading to improved PDT efficacy. [, ]
- Introduction of hydrophilic groups: Enhances water solubility and can improve tumor targeting. [, , ]
- Modification of the anhydride ring: Converting the anhydride ring to imide derivatives can influence singlet oxygen quantum yield, lipophilicity, and PDT efficacy. [, , ]
- Metal complexation: Complexation with metals like copper or zinc can alter photophysical properties and biological activity. [, , ]
- Dimerization: Formation of symmetrical or unsymmetrical dimers can influence absorption wavelengths, singlet oxygen quantum yields, and in vivo PDT efficacy. []
A:
- Stability: Generally stable in organic solvents but can be prone to degradation in biological environments. [, ]
- Formulation Strategies:
- Nanoparticles: Encapsulation in nanoparticles like liposomes, polymeric nanoparticles, and gold nanoparticles improves stability, enhances cellular uptake, and allows for controlled release. [, , , , , , , , , ]
- Nanofibers: Incorporation into PLLA nanofibers provides a sustained release system for PDT applications. []
A:
- Absorption: Primarily administered intravenously or intratumorally, but oral bioavailability can be limited. [, ]
- Distribution: Shows preferential accumulation in tumor tissues due to the enhanced permeability and retention (EPR) effect, but binding to serum albumin and lipoproteins can also influence its distribution. [, ]
- Metabolism: Information on specific metabolic pathways is limited. []
- Excretion: Excretion pathways have not been extensively studied. []
- In vivo activity: Demonstrates significant antitumor activity in various animal models, including cervical, prostate, pancreatic, and breast cancer models. [, , , ]
A:
A: While specific resistance mechanisms have not been extensively studied, overexpression of drug efflux transporters like P-glycoprotein (P-gp) could potentially limit its efficacy. []
A:
- Toxicity: Generally well-tolerated at therapeutic doses in preclinical studies. [, , ]
- Adverse effects: Potential for skin photosensitivity after administration. []
A:
- Nanoparticle encapsulation: Liposomes, polymeric nanoparticles, and gold nanoparticles improve targeted delivery, enhance cellular uptake, and allow for controlled release. [, , , , , , , , , ]
- Antibody conjugation: Conjugation to antibodies targeting tumor-specific antigens could improve selective delivery. []
- Ligand modification: Introducing ligands that bind to receptors overexpressed on cancer cells can enhance targeted accumulation. []
A:
- Fluorescence imaging: The inherent fluorescence of this compound and its derivatives allows for real-time monitoring of drug distribution and treatment response. [, , , ]
- Photoacoustic imaging: this compound can also be used as a contrast agent for photoacoustic imaging, providing deeper tissue penetration compared to fluorescence imaging. [, ]
A:
- UV-Vis Spectroscopy: Used to determine concentration and monitor reactions. [, , , ]
- Fluorescence Spectroscopy: Used to study interactions with biomolecules, characterize nanoparticles, and monitor drug delivery. [, , , ]
- NMR Spectroscopy: Provides detailed structural information and can be used to monitor reactions. [, , , ]
- Mass Spectrometry: Used for molecular weight determination and structural analysis. [, ]
- HPLC: Employed to separate and quantify this compound and its derivatives. []
Disclaimer and Information on In-Vitro Research Products
Please be aware that all articles and product information presented on BenchChem are intended solely for informational purposes. The products available for purchase on BenchChem are specifically designed for in-vitro studies, which are conducted outside of living organisms. In-vitro studies, derived from the Latin term "in glass," involve experiments performed in controlled laboratory settings using cells or tissues. It is important to note that these products are not categorized as medicines or drugs, and they have not received approval from the FDA for the prevention, treatment, or cure of any medical condition, ailment, or disease. We must emphasize that any form of bodily introduction of these products into humans or animals is strictly prohibited by law. It is essential to adhere to these guidelines to ensure compliance with legal and ethical standards in research and experimentation.