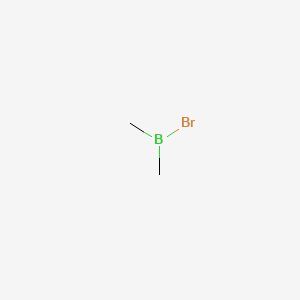
Bromodimethylborane
Overview
Description
Bromodimethylborane, also known as Dimethylboron bromide, is a chemical compound with the formula (CH3)2BBr . It has a molecular weight of 120.78 .
Molecular Structure Analysis
The linear formula of this compound is (CH3)2BBr . This indicates that the molecule consists of a boron atom bonded to two methyl groups (CH3) and a bromine atom .Physical And Chemical Properties Analysis
This compound is a liquid at room temperature . It has a boiling point of 31-32°C and a melting point of -129°C . The density of this compound is 1.238 g/mL at 25°C .Scientific Research Applications
Debenzylation and Detritylation
Bromodimethylborane is utilized in the synthesis of optically pure mono-acid or mixed-acid 1,2- or 2,3-diacyl-sn-glycerols, using a novel method for deprotecting primary alcohols. This method is particularly useful in synthesizing long saturated as well as unsaturated diacyl-sn-glycerols with minimal acyl migration during deprotection (Kodali & Duclos, 1992).
Cross-Coupling Reactions
Bromo-carboranes, related to this compound, have been successfully used in palladium-catalyzed cross-coupling for efficient B-N, B-O, and B-CN bond formation. These compounds are advantageous in such reactions, often outperforming traditional iodo-carborane species (Dziedzic et al., 2016).
Synthesis of Alkenes
This compound is used in the highly stereo- and regioselective synthesis of (Z)-trisubstituted alkenes. It is involved in hydroboration of 1-bromo-1-alkynes, facilitating Suzuki or Negishi coupling in the synthesis process (Huang & Negishi, 2007).
Luminescent Conjugated Organoboranes
The selective and differential reactivity of bromoboranes, including this compound, is critical in the preparation of luminescent fluoreneborane oligomers. This method reveals how the LUMO energy levels and quantum efficiency are affected by chain length in these organoboranes (Chen, Lalancette, & Jäkle, 2011).
Radical Addition Reactions
This compound is instrumental in bromine atom-transfer radical addition reactions in various solvents. It demonstrates the importance of solvent effects on the efficiency and course of these reactions, which are significant in organic chemistry (Yorimitsu et al., 2001).
Polymer Modification
In the modification of polymers, this compound plays a role in bromoalkylation processes. For instance, it is used in surface heparinization of polyurethane, demonstrating its versatility in enhancing polymer properties for biomedical applications (Alferiev et al., 2006).
Safety and Hazards
properties
IUPAC Name |
bromo(dimethyl)borane | |
---|---|---|
Source | PubChem | |
URL | https://pubchem.ncbi.nlm.nih.gov | |
Description | Data deposited in or computed by PubChem | |
InChI |
InChI=1S/C2H6BBr/c1-3(2)4/h1-2H3 | |
Source | PubChem | |
URL | https://pubchem.ncbi.nlm.nih.gov | |
Description | Data deposited in or computed by PubChem | |
InChI Key |
ABQPEYRVNHDPIO-UHFFFAOYSA-N | |
Source | PubChem | |
URL | https://pubchem.ncbi.nlm.nih.gov | |
Description | Data deposited in or computed by PubChem | |
Canonical SMILES |
B(C)(C)Br | |
Source | PubChem | |
URL | https://pubchem.ncbi.nlm.nih.gov | |
Description | Data deposited in or computed by PubChem | |
Molecular Formula |
C2H6BBr | |
Source | PubChem | |
URL | https://pubchem.ncbi.nlm.nih.gov | |
Description | Data deposited in or computed by PubChem | |
DSSTOX Substance ID |
DTXSID50199534 | |
Record name | Dimethylboronbromide | |
Source | EPA DSSTox | |
URL | https://comptox.epa.gov/dashboard/DTXSID50199534 | |
Description | DSSTox provides a high quality public chemistry resource for supporting improved predictive toxicology. | |
Molecular Weight |
120.79 g/mol | |
Source | PubChem | |
URL | https://pubchem.ncbi.nlm.nih.gov | |
Description | Data deposited in or computed by PubChem | |
CAS RN |
5158-50-9 | |
Record name | Dimethylboronbromide | |
Source | ChemIDplus | |
URL | https://pubchem.ncbi.nlm.nih.gov/substance/?source=chemidplus&sourceid=0005158509 | |
Description | ChemIDplus is a free, web search system that provides access to the structure and nomenclature authority files used for the identification of chemical substances cited in National Library of Medicine (NLM) databases, including the TOXNET system. | |
Record name | Dimethylboronbromide | |
Source | EPA DSSTox | |
URL | https://comptox.epa.gov/dashboard/DTXSID50199534 | |
Description | DSSTox provides a high quality public chemistry resource for supporting improved predictive toxicology. | |
Record name | Bromodimethylborane | |
Source | European Chemicals Agency (ECHA) | |
URL | https://echa.europa.eu/information-on-chemicals | |
Description | The European Chemicals Agency (ECHA) is an agency of the European Union which is the driving force among regulatory authorities in implementing the EU's groundbreaking chemicals legislation for the benefit of human health and the environment as well as for innovation and competitiveness. | |
Explanation | Use of the information, documents and data from the ECHA website is subject to the terms and conditions of this Legal Notice, and subject to other binding limitations provided for under applicable law, the information, documents and data made available on the ECHA website may be reproduced, distributed and/or used, totally or in part, for non-commercial purposes provided that ECHA is acknowledged as the source: "Source: European Chemicals Agency, http://echa.europa.eu/". Such acknowledgement must be included in each copy of the material. ECHA permits and encourages organisations and individuals to create links to the ECHA website under the following cumulative conditions: Links can only be made to webpages that provide a link to the Legal Notice page. | |
Record name | BROMODIMETHYLBORANE | |
Source | FDA Global Substance Registration System (GSRS) | |
URL | https://gsrs.ncats.nih.gov/ginas/app/beta/substances/EB60O8M22H | |
Description | The FDA Global Substance Registration System (GSRS) enables the efficient and accurate exchange of information on what substances are in regulated products. Instead of relying on names, which vary across regulatory domains, countries, and regions, the GSRS knowledge base makes it possible for substances to be defined by standardized, scientific descriptions. | |
Explanation | Unless otherwise noted, the contents of the FDA website (www.fda.gov), both text and graphics, are not copyrighted. They are in the public domain and may be republished, reprinted and otherwise used freely by anyone without the need to obtain permission from FDA. Credit to the U.S. Food and Drug Administration as the source is appreciated but not required. | |
Retrosynthesis Analysis
AI-Powered Synthesis Planning: Our tool employs the Template_relevance Pistachio, Template_relevance Bkms_metabolic, Template_relevance Pistachio_ringbreaker, Template_relevance Reaxys, Template_relevance Reaxys_biocatalysis model, leveraging a vast database of chemical reactions to predict feasible synthetic routes.
One-Step Synthesis Focus: Specifically designed for one-step synthesis, it provides concise and direct routes for your target compounds, streamlining the synthesis process.
Accurate Predictions: Utilizing the extensive PISTACHIO, BKMS_METABOLIC, PISTACHIO_RINGBREAKER, REAXYS, REAXYS_BIOCATALYSIS database, our tool offers high-accuracy predictions, reflecting the latest in chemical research and data.
Strategy Settings
Precursor scoring | Relevance Heuristic |
---|---|
Min. plausibility | 0.01 |
Model | Template_relevance |
Template Set | Pistachio/Bkms_metabolic/Pistachio_ringbreaker/Reaxys/Reaxys_biocatalysis |
Top-N result to add to graph | 6 |
Feasible Synthetic Routes
Disclaimer and Information on In-Vitro Research Products
Please be aware that all articles and product information presented on BenchChem are intended solely for informational purposes. The products available for purchase on BenchChem are specifically designed for in-vitro studies, which are conducted outside of living organisms. In-vitro studies, derived from the Latin term "in glass," involve experiments performed in controlled laboratory settings using cells or tissues. It is important to note that these products are not categorized as medicines or drugs, and they have not received approval from the FDA for the prevention, treatment, or cure of any medical condition, ailment, or disease. We must emphasize that any form of bodily introduction of these products into humans or animals is strictly prohibited by law. It is essential to adhere to these guidelines to ensure compliance with legal and ethical standards in research and experimentation.