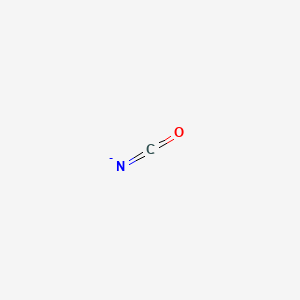
Cyanate
Overview
Description
Cyanate is an anion with the chemical formula OCN−. It is a resonance hybrid of three forms: [O−−C≡N] (61%), [O=C=N−] (30%), and [O+≡C−N2−] (4%) . This compound is derived from isocyanic acid (H−N=C=O) and its lesser tautomer cyanic acid (H−O−C≡N).
Preparation Methods
Cyanate can be synthesized through various methods:
Laboratory Synthesis: One common method involves the reaction of urea with sodium carbonate at high temperatures, producing sodium this compound, carbon dioxide, ammonia, and water . [ \text{Na}_2\text{CO}_3 + 2 \text{OC(NH}_2\text{)}_2 \rightarrow 2 \text{NaNCO} + \text{CO}_2 + 2 \text{NH}_3 + \text{H}_2\text{O} ]
Industrial Production: Industrially, cyanates are produced by oxidizing cyanides.
Chemical Reactions Analysis
Cyanate undergoes various chemical reactions:
Oxidation: Cyanates can be formed by the oxidation of cyanides.
Hydrolysis: This compound can hydrolyze to form ammonia and carbon dioxide, especially in biological systems .
Carbamylation: This compound can react with amino groups in proteins, leading to carbamylation, which can alter protein function and stability .
Scientific Research Applications
Cyanate has diverse applications in scientific research:
Mechanism of Action
The mechanism of action of cyanate involves its ability to carbamylate amino groups in proteins. This reaction can alter the protein’s conformation and function. For example, in sickle cell disease, this compound carbamylates the NH2-terminal residues of hemoglobin, increasing its oxygen affinity and preventing red cell sickling . This compound can also serve as a nitrogen source for microorganisms through the action of cyanase, which decomposes this compound into ammonia and carbon dioxide .
Comparison with Similar Compounds
Cyanate is similar to other nitrogen-containing compounds such as:
Isothis compound (−N=C=O): Unlike this compound, isothis compound is highly reactive and used in the production of polyurethanes.
Fulminate (−O−N+≡C−): Fulminate is much less stable than this compound and is known for its explosive properties.
Nitrile oxide (−CNO or −C≡N+−O−): Nitrile oxides are used in organic synthesis for cycloaddition reactions.
This compound’s unique properties, such as its ability to act as an ambidentate ligand and its role in biological nitrogen cycling, distinguish it from these similar compounds .
Properties
CAS No. |
661-20-1 |
---|---|
Molecular Formula |
CNO- |
Molecular Weight |
42.017 g/mol |
IUPAC Name |
isocyanate |
InChI |
InChI=1S/CNO/c2-1-3/q-1 |
InChI Key |
IQPQWNKOIGAROB-UHFFFAOYSA-N |
SMILES |
C(=[N-])=O |
Canonical SMILES |
C(=[N-])=O |
Origin of Product |
United States |
Synthesis routes and methods I
Procedure details
Synthesis routes and methods II
Procedure details
Synthesis routes and methods III
Procedure details
Synthesis routes and methods IV
Procedure details
Synthesis routes and methods V
Procedure details
Retrosynthesis Analysis
AI-Powered Synthesis Planning: Our tool employs the Template_relevance Pistachio, Template_relevance Bkms_metabolic, Template_relevance Pistachio_ringbreaker, Template_relevance Reaxys, Template_relevance Reaxys_biocatalysis model, leveraging a vast database of chemical reactions to predict feasible synthetic routes.
One-Step Synthesis Focus: Specifically designed for one-step synthesis, it provides concise and direct routes for your target compounds, streamlining the synthesis process.
Accurate Predictions: Utilizing the extensive PISTACHIO, BKMS_METABOLIC, PISTACHIO_RINGBREAKER, REAXYS, REAXYS_BIOCATALYSIS database, our tool offers high-accuracy predictions, reflecting the latest in chemical research and data.
Strategy Settings
Precursor scoring | Relevance Heuristic |
---|---|
Min. plausibility | 0.01 |
Model | Template_relevance |
Template Set | Pistachio/Bkms_metabolic/Pistachio_ringbreaker/Reaxys/Reaxys_biocatalysis |
Top-N result to add to graph | 6 |
Feasible Synthetic Routes
Disclaimer and Information on In-Vitro Research Products
Please be aware that all articles and product information presented on BenchChem are intended solely for informational purposes. The products available for purchase on BenchChem are specifically designed for in-vitro studies, which are conducted outside of living organisms. In-vitro studies, derived from the Latin term "in glass," involve experiments performed in controlled laboratory settings using cells or tissues. It is important to note that these products are not categorized as medicines or drugs, and they have not received approval from the FDA for the prevention, treatment, or cure of any medical condition, ailment, or disease. We must emphasize that any form of bodily introduction of these products into humans or animals is strictly prohibited by law. It is essential to adhere to these guidelines to ensure compliance with legal and ethical standards in research and experimentation.