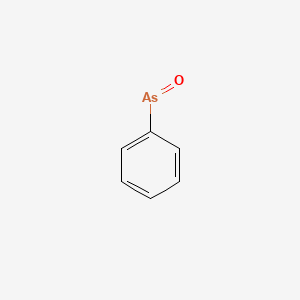
Phenylarsine oxide
Overview
Description
Phenylarsine oxide is an organometallic compound with the empirical formula C₆H₅AsO. It contains a phenyl group and an oxygen atom both bonded to an arsenic atom . Despite its simple empirical formula, this compound does not contain an arsenic-oxygen double bond. Instead, it forms a cyclic oligomer, typically crystallizing as a tetramer .
Mechanism of Action
Target of Action
Phenylarsine oxide (PAO) is an organometallic compound with the empirical formula C6H5AsO . It primarily targets the sulfur atom of thiols in organic compounds, particularly forming stable complexes with vicinal cysteine residues in protein structures . This makes it useful for studying ligand-receptor binding . PAO also inhibits the internalization of cell surface receptors and tyrosine phosphatases, without affecting tyrosine kinase .
Mode of Action
PAO interacts with its targets by forming stable complexes with vicinal cysteine residues in protein structures . This interaction inhibits the internalization of cell surface receptors and tyrosine phosphatases . Instead, it forms a cyclic oligomer, with a range of possible ring sizes .
Biochemical Pathways
PAO affects several biochemical pathways. It stimulates mitochondrial ion fluxes , which can influence energy production within cells. PAO also induces apoptosis in HepG2 cells via ROS-mediated mitochondria and ER-stress dependent signaling pathways . Furthermore, PAO can reduce the motility and sustainability of Setaria cervi .
Pharmacokinetics
It is known that pao can inhibit clathrin-dependent endocytosis, a process that could affect the absorption, distribution, metabolism, and excretion (adme) of the compound .
Result of Action
PAO has potent cytotoxic effects against human hepatocellular carcinoma (HCC) HepG2 cells . It activates apoptosis-related proteins in a dose- and time-dependent manner . PAO also stimulates 2-deoxyglucose transport in insulin-resistant human skeletal muscle and activates p56 lck protein tyrosine kinase .
Action Environment
PAO is a reducing agent that is stable in water . This stability allows it to be used in iodometric methods for the determination of residual chlorine in wastewaters . It is very toxic to aquatic organisms and may cause long-term adverse effects in the aquatic environment . Therefore, the environmental factors can significantly influence the action, efficacy, and stability of PAO.
Biochemical Analysis
Biochemical Properties
Phenylarsine oxide plays a significant role in biochemical reactions due to its ability to form stable complexes with vicinal cysteine residues in protein structures. This property makes it useful for studying ligand-receptor binding and for affinity chromatography by immobilizing it on a resin . This compound inhibits tyrosine phosphatases without affecting tyrosine kinase activity, making it a metabolic poison . It also stimulates mitochondrial ion fluxes and inhibits the internalization of cell surface receptors .
Cellular Effects
This compound has been shown to induce apoptosis in various cell types, including human hepatocellular carcinoma (HepG2) cells. It activates apoptosis-related proteins such as caspase-3 and -9 and poly-ADP ribose polymerase in a dose- and time-dependent manner . This compound also increases intracellular reactive oxygen species (ROS) specifically in the mitochondria and endoplasmic reticulum, leading to cytotoxicity . Additionally, it inhibits cell proliferation and induces apoptosis in Bax- and Bak-deficient cancer cells .
Molecular Mechanism
At the molecular level, this compound exerts its effects by binding to vicinal sulfhydryl groups of proteins, forming stable ring structures . This binding inhibits the action of many thiol-containing enzymes and affects human endothelial cell permeability through the induction of occludin proteolysis . This compound also elevates intracellular calcium levels in thymocytes and inhibits Ca²⁺-dependent ATPase activity in both thymocytes and sarcoplasmic reticulum from skeletal muscle .
Temporal Effects in Laboratory Settings
In laboratory settings, the effects of this compound change over time. It has been observed to induce apoptosis in a time-dependent manner, with intracellular ROS accumulating in the mitochondria and endoplasmic reticulum after exposure . The stability and degradation of this compound in laboratory settings have not been extensively studied, but its long-term effects on cellular function include sustained cytotoxicity and apoptosis .
Dosage Effects in Animal Models
The effects of this compound vary with different dosages in animal models. At higher doses, this compound induces significant cytotoxicity and apoptosis in cancer cells . In murine models, this compound has been shown to cause dose-dependent upregulation of ROS, cytokines, and unfolded protein response (UPR) proteins, leading to inflammation and apoptosis . Toxic or adverse effects at high doses include severe cytotoxicity and potential damage to normal tissues .
Metabolic Pathways
This compound is involved in metabolic pathways that include the inhibition of protein tyrosine phosphatases and the induction of ROS-mediated signaling pathways . It binds with cysteine residues of intracellular proteins, affecting metabolic flux and metabolite levels . This compound also interacts with enzymes involved in the regulation of intracellular calcium levels and ATPase activity .
Transport and Distribution
This compound is transported and distributed within cells and tissues through passive diffusion . It interacts with transporters and binding proteins that facilitate its localization and accumulation in specific cellular compartments . This compound’s high affinity for sulfur atoms in thiols allows it to form stable complexes with proteins, affecting its distribution within cells .
Subcellular Localization
This compound localizes to the mitochondria and endoplasmic reticulum, where it induces ROS-mediated cytotoxicity . It also affects the plasma membrane by inhibiting the internalization of cell surface receptors . The subcellular localization of this compound is influenced by its ability to bind to vicinal sulfhydryl groups and form stable complexes with proteins .
Preparation Methods
Phenylarsine oxide can be synthesized through various methods. One common synthetic route involves the reaction of phenylarsine dichloride with water, which results in the formation of this compound and hydrochloric acid . The reaction conditions typically involve the use of an inert atmosphere to prevent oxidation and the use of a solvent such as benzene or toluene to dissolve the reactants.
Chemical Reactions Analysis
Phenylarsine oxide undergoes several types of chemical reactions, including:
Oxidation: this compound can be oxidized to form phenylarsinic acid.
Reduction: It can be reduced to phenylarsine.
Substitution: This compound can undergo substitution reactions with various nucleophiles, such as thiols, to form stable complexes.
Common reagents used in these reactions include oxidizing agents like hydrogen peroxide for oxidation, reducing agents like sodium borohydride for reduction, and nucleophiles like thiols for substitution reactions. The major products formed from these reactions include phenylarsinic acid, phenylarsine, and various thiol-phenylarsine oxide complexes .
Scientific Research Applications
Phenylarsine oxide has several scientific research applications:
Affinity Chromatography: This compound is immobilized on a resin for affinity chromatography, selectively binding structures with vicinal cysteines.
Wastewater Analysis: It is used as a reducing agent in iodometric methods for determining residual chlorine in wastewaters.
Electrochemical Sensors: This compound is used in the development of sensors for detecting arsenic in drinking water.
Comparison with Similar Compounds
Phenylarsine oxide is unique among arsenic compounds due to its ability to form stable complexes with vicinal thiols. Similar compounds include:
Phenylarsenic acid: Contains an arsenic-oxygen double bond and does not form cyclic oligomers.
Phenylarsine dichloride: Used as a precursor in the synthesis of this compound.
Arsenobenzene: Another organoarsenic compound, but with different reactivity and applications.
This compound’s unique ability to form stable complexes with vicinal thiols makes it particularly useful in biochemical research and affinity chromatography .
Properties
IUPAC Name |
arsorosobenzene | |
---|---|---|
Source | PubChem | |
URL | https://pubchem.ncbi.nlm.nih.gov | |
Description | Data deposited in or computed by PubChem | |
InChI |
InChI=1S/C6H5AsO/c8-7-6-4-2-1-3-5-6/h1-5H | |
Source | PubChem | |
URL | https://pubchem.ncbi.nlm.nih.gov | |
Description | Data deposited in or computed by PubChem | |
InChI Key |
BQVCCPGCDUSGOE-UHFFFAOYSA-N | |
Source | PubChem | |
URL | https://pubchem.ncbi.nlm.nih.gov | |
Description | Data deposited in or computed by PubChem | |
Canonical SMILES |
C1=CC=C(C=C1)[As]=O | |
Source | PubChem | |
URL | https://pubchem.ncbi.nlm.nih.gov | |
Description | Data deposited in or computed by PubChem | |
Molecular Formula |
C6H5AsO | |
Source | PubChem | |
URL | https://pubchem.ncbi.nlm.nih.gov | |
Description | Data deposited in or computed by PubChem | |
DSSTOX Substance ID |
DTXSID5042249 | |
Record name | Phenylarsine oxide | |
Source | EPA DSSTox | |
URL | https://comptox.epa.gov/dashboard/DTXSID5042249 | |
Description | DSSTox provides a high quality public chemistry resource for supporting improved predictive toxicology. | |
Molecular Weight |
168.02 g/mol | |
Source | PubChem | |
URL | https://pubchem.ncbi.nlm.nih.gov | |
Description | Data deposited in or computed by PubChem | |
Physical Description |
Crystals with a melting point of 144-146 degrees C; [Merck Index] | |
Record name | Phenyl arsine oxide | |
Source | Haz-Map, Information on Hazardous Chemicals and Occupational Diseases | |
URL | https://haz-map.com/Agents/1912 | |
Description | Haz-Map® is an occupational health database designed for health and safety professionals and for consumers seeking information about the adverse effects of workplace exposures to chemical and biological agents. | |
Explanation | Copyright (c) 2022 Haz-Map(R). All rights reserved. Unless otherwise indicated, all materials from Haz-Map are copyrighted by Haz-Map(R). No part of these materials, either text or image may be used for any purpose other than for personal use. Therefore, reproduction, modification, storage in a retrieval system or retransmission, in any form or by any means, electronic, mechanical or otherwise, for reasons other than personal use, is strictly prohibited without prior written permission. | |
CAS No. |
637-03-6 | |
Record name | Phenylarsine oxide | |
Source | CAS Common Chemistry | |
URL | https://commonchemistry.cas.org/detail?cas_rn=637-03-6 | |
Description | CAS Common Chemistry is an open community resource for accessing chemical information. Nearly 500,000 chemical substances from CAS REGISTRY cover areas of community interest, including common and frequently regulated chemicals, and those relevant to high school and undergraduate chemistry classes. This chemical information, curated by our expert scientists, is provided in alignment with our mission as a division of the American Chemical Society. | |
Explanation | The data from CAS Common Chemistry is provided under a CC-BY-NC 4.0 license, unless otherwise stated. | |
Record name | Oxophenylarsine | |
Source | ChemIDplus | |
URL | https://pubchem.ncbi.nlm.nih.gov/substance/?source=chemidplus&sourceid=0000637036 | |
Description | ChemIDplus is a free, web search system that provides access to the structure and nomenclature authority files used for the identification of chemical substances cited in National Library of Medicine (NLM) databases, including the TOXNET system. | |
Record name | phenylarsine oxide | |
Source | DTP/NCI | |
URL | https://dtp.cancer.gov/dtpstandard/servlet/dwindex?searchtype=NSC&outputformat=html&searchlist=42470 | |
Description | The NCI Development Therapeutics Program (DTP) provides services and resources to the academic and private-sector research communities worldwide to facilitate the discovery and development of new cancer therapeutic agents. | |
Explanation | Unless otherwise indicated, all text within NCI products is free of copyright and may be reused without our permission. Credit the National Cancer Institute as the source. | |
Record name | Arsine, oxophenyl- | |
Source | EPA Chemicals under the TSCA | |
URL | https://www.epa.gov/chemicals-under-tsca | |
Description | EPA Chemicals under the Toxic Substances Control Act (TSCA) collection contains information on chemicals and their regulations under TSCA, including non-confidential content from the TSCA Chemical Substance Inventory and Chemical Data Reporting. | |
Record name | Phenylarsine oxide | |
Source | EPA DSSTox | |
URL | https://comptox.epa.gov/dashboard/DTXSID5042249 | |
Description | DSSTox provides a high quality public chemistry resource for supporting improved predictive toxicology. | |
Record name | Phenylarsine oxide | |
Source | European Chemicals Agency (ECHA) | |
URL | https://echa.europa.eu/substance-information/-/substanceinfo/100.010.251 | |
Description | The European Chemicals Agency (ECHA) is an agency of the European Union which is the driving force among regulatory authorities in implementing the EU's groundbreaking chemicals legislation for the benefit of human health and the environment as well as for innovation and competitiveness. | |
Explanation | Use of the information, documents and data from the ECHA website is subject to the terms and conditions of this Legal Notice, and subject to other binding limitations provided for under applicable law, the information, documents and data made available on the ECHA website may be reproduced, distributed and/or used, totally or in part, for non-commercial purposes provided that ECHA is acknowledged as the source: "Source: European Chemicals Agency, http://echa.europa.eu/". Such acknowledgement must be included in each copy of the material. ECHA permits and encourages organisations and individuals to create links to the ECHA website under the following cumulative conditions: Links can only be made to webpages that provide a link to the Legal Notice page. | |
Record name | OXOPHENYLARSINE | |
Source | FDA Global Substance Registration System (GSRS) | |
URL | https://gsrs.ncats.nih.gov/ginas/app/beta/substances/0HUR2WY345 | |
Description | The FDA Global Substance Registration System (GSRS) enables the efficient and accurate exchange of information on what substances are in regulated products. Instead of relying on names, which vary across regulatory domains, countries, and regions, the GSRS knowledge base makes it possible for substances to be defined by standardized, scientific descriptions. | |
Explanation | Unless otherwise noted, the contents of the FDA website (www.fda.gov), both text and graphics, are not copyrighted. They are in the public domain and may be republished, reprinted and otherwise used freely by anyone without the need to obtain permission from FDA. Credit to the U.S. Food and Drug Administration as the source is appreciated but not required. | |
Retrosynthesis Analysis
AI-Powered Synthesis Planning: Our tool employs the Template_relevance Pistachio, Template_relevance Bkms_metabolic, Template_relevance Pistachio_ringbreaker, Template_relevance Reaxys, Template_relevance Reaxys_biocatalysis model, leveraging a vast database of chemical reactions to predict feasible synthetic routes.
One-Step Synthesis Focus: Specifically designed for one-step synthesis, it provides concise and direct routes for your target compounds, streamlining the synthesis process.
Accurate Predictions: Utilizing the extensive PISTACHIO, BKMS_METABOLIC, PISTACHIO_RINGBREAKER, REAXYS, REAXYS_BIOCATALYSIS database, our tool offers high-accuracy predictions, reflecting the latest in chemical research and data.
Strategy Settings
Precursor scoring | Relevance Heuristic |
---|---|
Min. plausibility | 0.01 |
Model | Template_relevance |
Template Set | Pistachio/Bkms_metabolic/Pistachio_ringbreaker/Reaxys/Reaxys_biocatalysis |
Top-N result to add to graph | 6 |
Feasible Synthetic Routes
Q1: What is the primary mechanism of action of PAO?
A1: PAO exerts its effects primarily by binding to vicinal dithiol groups found in proteins. This interaction often leads to the formation of stable ring complexes, disrupting the normal function of the target protein. [, , , ]
Q2: How does PAO impact signal transduction pathways?
A2: PAO has been shown to affect several signaling pathways by inhibiting protein tyrosine phosphatases (PTPs). PTPs play a crucial role in regulating tyrosine phosphorylation, a key mechanism in signal transduction. By inhibiting PTPs, PAO can lead to increased tyrosine phosphorylation of signaling molecules, ultimately affecting cellular responses. [, , , ]
Q3: Can you provide specific examples of proteins affected by PAO?
A3: Research has identified several proteins targeted by PAO, including:
- Insulin receptor signaling: PAO disrupts insulin-stimulated glucose uptake by interfering with signal transmission from the insulin receptor, possibly by targeting a 15-kDa protein involved in this pathway. [, , ]
- Endothelial nitric oxide synthase (eNOS): PAO induces Ca2+-independent activation of eNOS, potentially through conformational changes or altered protein-protein interactions. []
- Tau protein: PAO increases tau phosphorylation within its microtubule-binding domain at specific non-Ser/Thr-Pro sites, leading to decreased microtubule stability. [, ]
- NADPH oxidase: PAO inhibits the activation of NADPH oxidase in neutrophils, primarily by binding to the beta subunit of the flavocytochrome b component. []
Q4: What is the molecular formula and weight of PAO?
A4: The molecular formula of PAO is C6H5AsO. Its molecular weight is 168.01 g/mol.
Q5: Is there any spectroscopic data available for PAO?
A5: While the provided research papers primarily focus on the biological effects of PAO, spectroscopic data like NMR or IR spectra are not extensively discussed.
Q6: Are there any specific applications of PAO related to its material properties?
A8: The provided research primarily focuses on the biological applications of PAO. While it has historical uses in chemical synthesis [], the papers do not delve into material-specific applications.
Q7: Does PAO exhibit any catalytic properties?
A7: PAO is not typically known for its catalytic properties. Its primary mode of action involves binding to and inhibiting target proteins rather than catalyzing chemical reactions.
Q8: What are the main findings from in vitro studies using PAO?
A8: In vitro studies have demonstrated the ability of PAO to:
- Inhibit insulin-stimulated glucose uptake in adipocytes [, , ]
- Activate eNOS in endothelial cells []
- Modulate tau phosphorylation and microtubule stability in neuronal cells [, ]
- Inhibit NADPH oxidase activation in neutrophils []
Q9: What insights have been gained from in vivo studies using PAO?
A9: In vivo studies using PAO have provided evidence for its role in:
- Regulating cerebral vascular tone in newborn pigs []
- Modulating cardiac sympathetic afferent reflex in rats []
Q10: Are there specific drug delivery strategies being explored for PAO?
A17: While the research primarily uses PAO as a tool to study cellular mechanisms, some studies explore its potential therapeutic applications. For instance, researchers have investigated magnetic poly D,L-lactide-co-glycolide PAO nanoparticles (M-PLGA-PAO-NS) for targeted delivery and inhibition of hepatic carcinoma. []
Q11: What analytical techniques are commonly used to study PAO?
A11: The research papers employ various techniques to investigate the effects of PAO, including:
- Radioligand binding assays: To study the interaction of PAO with specific proteins, such as the dopamine transporter. []
- Immunoblotting: To analyze changes in protein phosphorylation levels upon PAO treatment. [, , ]
- Enzyme activity assays: To assess the impact of PAO on the activity of enzymes like eNOS, NADPH oxidase, and PTPs. [, , ]
- Cell-based assays: To evaluate the effects of PAO on cellular processes such as glucose uptake, cell death, and signal transduction. [, , , ]
- Animal models: To investigate the physiological effects of PAO in vivo. [, ]
Q12: Are there any specific challenges in analyzing PAO?
A20: Analyzing PAO can be challenging due to its reactivity and potential for non-specific interactions. Researchers often employ careful controls and specific analytical methods to ensure accurate and reliable results. For example, using the dithiol compound 2,3-dimercaptopropanol to reverse PAO's effects helps confirm the specificity of its action on vicinal dithiols. [, ]
Q13: What is known about the environmental impact of PAO?
A21: The research papers primarily focus on the biochemical and cellular effects of PAO. While its use in specific applications, such as industrial cooling waters, is mentioned [], the broader environmental impact and degradation pathways are not extensively discussed.
Disclaimer and Information on In-Vitro Research Products
Please be aware that all articles and product information presented on BenchChem are intended solely for informational purposes. The products available for purchase on BenchChem are specifically designed for in-vitro studies, which are conducted outside of living organisms. In-vitro studies, derived from the Latin term "in glass," involve experiments performed in controlled laboratory settings using cells or tissues. It is important to note that these products are not categorized as medicines or drugs, and they have not received approval from the FDA for the prevention, treatment, or cure of any medical condition, ailment, or disease. We must emphasize that any form of bodily introduction of these products into humans or animals is strictly prohibited by law. It is essential to adhere to these guidelines to ensure compliance with legal and ethical standards in research and experimentation.