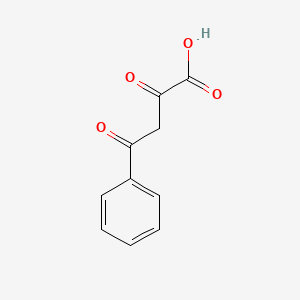
2,4-Dioxo-4-phenylbutanoic acid
Overview
Description
2,4-Dioxo-4-phenylbutanoic acid is an organic compound with the molecular formula C10H8O4. It is characterized by the presence of two carbonyl groups and a phenyl group attached to a four-carbon chain.
Mechanism of Action
Target of Action
The primary target of 2,4-Dioxo-4-phenylbutanoic acid (DPBA) is the viral polymerase of the influenza virus . This enzyme performs transcription and replication of the RNA genome, making it an attractive target for antiviral drugs .
Mode of Action
DPBA acts as an inhibitor of the influenza virus by binding to its surface proteins . It specifically inhibits the cap-snatching mechanism of the viral polymerase, which is essential for the transcription of the virus .
Biochemical Pathways
The cap-snatching mechanism is a process where the viral polymerase “snatches” the cap from host pre-mRNAs, allowing the viral mRNAs to be recognized and bound by the host ribosome . By inhibiting this process, DPBA prevents the transcription and subsequent replication of the virus .
Result of Action
The inhibition of the cap-snatching mechanism by DPBA results in the prevention of viral mRNA transcription, thereby blocking the replication of the influenza virus . This could potentially lead to a decrease in the severity and duration of an influenza infection.
Biochemical Analysis
Biochemical Properties
2,4-Dioxo-4-phenylbutanoic acid plays a significant role in biochemical reactions, particularly as an inhibitor of viral polymerases. It interacts with enzymes such as the RNA-dependent RNA polymerase of the influenza virus, inhibiting the cap-snatching mechanism essential for viral transcription. This interaction prevents the virus from replicating its RNA genome, thereby inhibiting its proliferation. Additionally, this compound has been shown to inhibit nucleases and stabilize proteins at higher temperatures .
Cellular Effects
The effects of this compound on various cell types and cellular processes are profound. It influences cell function by inhibiting viral replication, which can lead to reduced viral load in infected cells . This compound also affects cell signaling pathways and gene expression by interfering with the transcriptional machinery of the virus. Furthermore, it has been observed to impact cellular metabolism by altering the normal metabolic flux in infected cells .
Molecular Mechanism
At the molecular level, this compound exerts its effects through specific binding interactions with viral polymerases. It inhibits the cap-snatching mechanism of the influenza virus polymerase, which is crucial for the transcription of viral RNA. This inhibition prevents the virus from synthesizing its RNA, thereby halting its replication. Additionally, this compound has been shown to bind to other viral surface proteins, further inhibiting viral activity .
Temporal Effects in Laboratory Settings
In laboratory settings, the effects of this compound have been observed to change over time. The compound is stable under normal conditions but may degrade under extreme conditions . Long-term studies have shown that it maintains its inhibitory effects on viral replication over extended periods, although its efficacy may decrease with prolonged exposure to high temperatures or reactive environments .
Dosage Effects in Animal Models
The effects of this compound vary with different dosages in animal models. At lower doses, it effectively inhibits viral replication without causing significant adverse effects . At higher doses, toxic effects such as cellular toxicity and metabolic disturbances have been observed . These threshold effects highlight the importance of optimizing dosage to maximize therapeutic benefits while minimizing adverse effects.
Metabolic Pathways
This compound is involved in metabolic pathways related to viral replication. It interacts with enzymes such as RNA-dependent RNA polymerase, inhibiting the transcription and replication of viral RNA. This interaction affects the overall metabolic flux within infected cells, leading to altered levels of metabolites and disruption of normal cellular processes .
Transport and Distribution
Within cells and tissues, this compound is transported and distributed through interactions with transporters and binding proteins . These interactions facilitate its localization to specific cellular compartments where it exerts its inhibitory effects on viral replication. The compound’s distribution is influenced by its chemical properties, such as solubility and stability .
Subcellular Localization
The subcellular localization of this compound is critical for its activity and function. It is directed to specific compartments within the cell, such as the nucleus and cytoplasm, where it interacts with viral polymerases and other biomolecules . Post-translational modifications and targeting signals play a role in directing the compound to these compartments, ensuring its effective inhibition of viral replication .
Preparation Methods
Synthetic Routes and Reaction Conditions
2,4-Dioxo-4-phenylbutanoic acid can be synthesized through several methods. One common approach involves the reaction of diethyl oxalate with acetophenone in the presence of a base, such as sodium ethoxide. The reaction proceeds through a Claisen condensation, followed by hydrolysis and decarboxylation to yield the desired product .
Industrial Production Methods
While specific industrial production methods for this compound are not extensively documented, the compound can be produced on a larger scale using similar synthetic routes as those employed in laboratory settings. Optimization of reaction conditions, such as temperature, solvent, and catalyst, can enhance yield and purity for industrial applications .
Chemical Reactions Analysis
Types of Reactions
2,4-Dioxo-4-phenylbutanoic acid undergoes various chemical reactions, including:
Oxidation: The compound can be oxidized to form corresponding carboxylic acids or ketones.
Reduction: Reduction reactions can convert the carbonyl groups to alcohols.
Substitution: The phenyl group can undergo electrophilic aromatic substitution reactions.
Common Reagents and Conditions
Oxidation: Common oxidizing agents include potassium permanganate (KMnO4) and chromium trioxide (CrO3).
Reduction: Reducing agents such as sodium borohydride (NaBH4) or lithium aluminum hydride (LiAlH4) are typically used.
Substitution: Electrophilic aromatic substitution reactions often involve reagents like bromine (Br2) or nitric acid (HNO3).
Major Products Formed
Oxidation: Carboxylic acids or ketones.
Reduction: Alcohols.
Substitution: Halogenated or nitrated aromatic compounds.
Scientific Research Applications
2,4-Dioxo-4-phenylbutanoic acid has several scientific research applications:
Comparison with Similar Compounds
2,4-Dioxo-4-phenylbutanoic acid can be compared with other similar compounds, such as:
Benzoyl pyruvic acid: Similar in structure but lacks the additional carbonyl group.
Diphenylacetic acid: Contains two phenyl groups but differs in the carbonyl group arrangement.
The uniqueness of this compound lies in its dual carbonyl groups and phenyl group, which confer distinct chemical reactivity and biological activity .
Properties
IUPAC Name |
2,4-dioxo-4-phenylbutanoic acid | |
---|---|---|
Source | PubChem | |
URL | https://pubchem.ncbi.nlm.nih.gov | |
Description | Data deposited in or computed by PubChem | |
InChI |
InChI=1S/C10H8O4/c11-8(6-9(12)10(13)14)7-4-2-1-3-5-7/h1-5H,6H2,(H,13,14) | |
Source | PubChem | |
URL | https://pubchem.ncbi.nlm.nih.gov | |
Description | Data deposited in or computed by PubChem | |
InChI Key |
JGKFWCXVYCDKDU-UHFFFAOYSA-N | |
Source | PubChem | |
URL | https://pubchem.ncbi.nlm.nih.gov | |
Description | Data deposited in or computed by PubChem | |
Canonical SMILES |
C1=CC=C(C=C1)C(=O)CC(=O)C(=O)O | |
Source | PubChem | |
URL | https://pubchem.ncbi.nlm.nih.gov | |
Description | Data deposited in or computed by PubChem | |
Molecular Formula |
C10H8O4 | |
Source | PubChem | |
URL | https://pubchem.ncbi.nlm.nih.gov | |
Description | Data deposited in or computed by PubChem | |
Molecular Weight |
192.17 g/mol | |
Source | PubChem | |
URL | https://pubchem.ncbi.nlm.nih.gov | |
Description | Data deposited in or computed by PubChem | |
Synthesis routes and methods
Procedure details
Retrosynthesis Analysis
AI-Powered Synthesis Planning: Our tool employs the Template_relevance Pistachio, Template_relevance Bkms_metabolic, Template_relevance Pistachio_ringbreaker, Template_relevance Reaxys, Template_relevance Reaxys_biocatalysis model, leveraging a vast database of chemical reactions to predict feasible synthetic routes.
One-Step Synthesis Focus: Specifically designed for one-step synthesis, it provides concise and direct routes for your target compounds, streamlining the synthesis process.
Accurate Predictions: Utilizing the extensive PISTACHIO, BKMS_METABOLIC, PISTACHIO_RINGBREAKER, REAXYS, REAXYS_BIOCATALYSIS database, our tool offers high-accuracy predictions, reflecting the latest in chemical research and data.
Strategy Settings
Precursor scoring | Relevance Heuristic |
---|---|
Min. plausibility | 0.01 |
Model | Template_relevance |
Template Set | Pistachio/Bkms_metabolic/Pistachio_ringbreaker/Reaxys/Reaxys_biocatalysis |
Top-N result to add to graph | 6 |
Feasible Synthetic Routes
Disclaimer and Information on In-Vitro Research Products
Please be aware that all articles and product information presented on BenchChem are intended solely for informational purposes. The products available for purchase on BenchChem are specifically designed for in-vitro studies, which are conducted outside of living organisms. In-vitro studies, derived from the Latin term "in glass," involve experiments performed in controlled laboratory settings using cells or tissues. It is important to note that these products are not categorized as medicines or drugs, and they have not received approval from the FDA for the prevention, treatment, or cure of any medical condition, ailment, or disease. We must emphasize that any form of bodily introduction of these products into humans or animals is strictly prohibited by law. It is essential to adhere to these guidelines to ensure compliance with legal and ethical standards in research and experimentation.