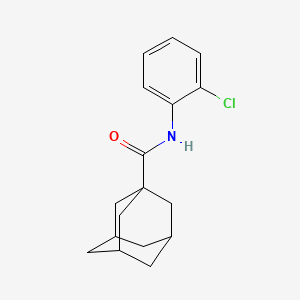
N-(2-chlorophenyl)adamantane-1-carboxamide
- Click on QUICK INQUIRY to receive a quote from our team of experts.
- With the quality product at a COMPETITIVE price, you can focus more on your research.
Overview
Description
N-(2-Chlorophenyl)adamantane-1-carboxamide is a synthetic compound featuring a rigid adamantane core linked to a carboxamide group and a 2-chlorophenyl substituent. The adamantane moiety imparts high lipophilicity and metabolic stability, while the 2-chlorophenyl group introduces electronic effects (e.g., electron-withdrawing) and steric bulk.
Preparation Methods
Synthetic Routes and Reaction Conditions
N-(2-chlorophenyl)adamantane-1-carboxamide can be synthesized through a one-step procedure by reacting adamantane-1-carboxylic acid with 2-chloroaniline in the presence of phosphorus trichloride, 4-dimethylaminopyridine (DMAP), and triethylamine. The reaction is typically carried out in acetonitrile under reflux conditions for about 8 hours. The yields of the target amides can range from 54% to 87% .
Industrial Production Methods
While specific industrial production methods for this compound are not well-documented, the general approach involves scaling up the laboratory synthesis methods. This includes optimizing reaction conditions, using continuous flow reactors, and ensuring the availability of high-purity starting materials to achieve consistent product quality.
Chemical Reactions Analysis
Key Reaction Steps
Step 1: Activation of Adamantane-1-Carboxylic Acid
-
CDI Method : The carboxylic acid reacts with CDI in a solvent like dichloromethane or dimethylformamide (DMF) to form an imidazolide intermediate .
Adamantane-1-carboxylic acid+CDI→Active intermediate -
EDC/HOAt Method : Adamantane-1-carboxylic acid is treated with EDC and 1-hydroxy-7-azabenzotriazole (HOAt) in the presence of a base (e.g., DIPEA) .
Step 2: Coupling with 2-Chloroaniline
-
The activated intermediate reacts with 2-chloroaniline in a solvent like DMF or dichloromethane. The reaction is typically stirred at room temperature or under reflux to yield the amide .
Active intermediate+2-Chloroaniline→This compound
Reaction Mechanism
The coupling process involves nucleophilic attack of the amine (2-chloroaniline) on the activated carbonyl group of the adamantane derivative. CDI facilitates this by forming a more electrophilic intermediate, while EDC/HOAt systems reduce side reactions (e.g., hydrolysis) by stabilizing the activated species .
Characterization Techniques
-
NMR Analysis :
-
X-ray Crystallography : Confirms molecular conformation and intermolecular interactions (e.g., hydrogen bonding) .
Data Table: Comparative Analysis of Coupling Agents
Challenges and Variations
-
Steric Hindrance : The bulky adamantane group can slow reaction rates, necessitating prolonged reaction times or elevated temperatures .
-
Alternative Substrates : Hydrazine derivatives or isothiocyanates may replace amines, leading to hydrazides or thioamides (e.g., as in ).
-
Functional Group Compatibility : Chlorine substituents on the phenyl ring may require careful control of reaction conditions to avoid side reactions .
Scientific Research Applications
Antiviral Activity
One of the most notable applications of N-(2-chlorophenyl)adamantane-1-carboxamide is its potential as an antiviral agent. Research indicates that derivatives of this compound exhibit significant inhibitory effects against human adenovirus (HAdV). For instance, certain analogues have demonstrated sub-micromolar potency and high selectivity indexes, making them promising candidates for treating infections in immunocompromised patients .
Case Study: Mechanism of Action
In a study investigating the antiviral properties of substituted phenyl derivatives, it was found that compounds targeting the HAdV DNA replication process showed enhanced activity compared to traditional treatments like niclosamide. Specifically, one derivative exhibited an IC50 value of 0.27 µM, indicating its effectiveness while maintaining low cytotoxicity .
Anticancer Properties
This compound has also been evaluated for its anticancer properties. In vitro studies have shown that related compounds exhibit potent antiproliferative activity against various cancer cell lines, including prostate, colorectal, and breast cancer cells. The compounds demonstrated IC50 values below 10 µM against HeLa and MCF-7 cell lines, suggesting strong potential for further development in cancer therapeutics .
Data Table: Antiproliferative Activity
Compound | Cell Line | IC50 (µM) |
---|---|---|
Compound A | HeLa | <10 |
Compound B | MCF-7 | <10 |
Compound C | HCT-116 | Moderate |
Compound D | HepG-2 | Moderate |
Compound E | PC-3 | Weak |
Enzyme Inhibition
The compound has been investigated for its role as an inhibitor of specific enzymes, particularly 11β-hydroxysteroid dehydrogenase type 1 (11β-HSD1). Docking studies suggest that this compound exhibits favorable binding interactions with this enzyme, which is crucial for regulating cortisol levels in the body .
Case Study: Binding Affinity
In a study focused on enzyme inhibition, the binding affinity of this compound was assessed through molecular docking simulations. The results indicated strong interactions with the enzyme's active site, supporting its potential as a therapeutic agent for conditions related to cortisol dysregulation.
Synthesis and Structural Analysis
The synthesis of this compound involves multi-step reactions that can be optimized for yield and purity. Recent advancements in synthetic methodologies have improved the efficiency of producing this compound, allowing for more extensive biological testing and application development .
Synthesis Overview
- Starting Materials: Adamantane derivatives and chlorinated phenyl compounds.
- Reaction Conditions: Typically involves coupling reactions under controlled temperature and pressure.
- Yield Improvement: Microwave-assisted synthesis has been shown to enhance yields significantly compared to traditional methods.
Mechanism of Action
The mechanism of action of N-(2-chlorophenyl)adamantane-1-carboxamide involves its interaction with specific molecular targets. For instance, in antiviral research, it has been shown to inhibit the entry of viruses into host cells by targeting viral glycoproteins. The compound’s rigid structure allows it to fit into specific binding sites, blocking the interaction between the virus and the host cell .
Comparison with Similar Compounds
Substituent Effects on Electronic Properties (35Cl NQR Analysis)
highlights the use of 35Cl nuclear quadrupole resonance (NQR) to study electronic environments in substituted amides. Key findings include:
- N-(2-Chlorophenyl)acetamide : The 35Cl NQR frequency is influenced by alkyl side chains, which lower the frequency, while aryl or chloroalkyl groups increase it.
- N-(2,6-Dichlorophenyl)adamantane-1-carboxamide : Additional chlorine substituents may further enhance electron withdrawal, amplifying NQR shifts .
Table 1: 35Cl NQR Frequencies and Substituent Effects
Compound | Substituent | 35Cl NQR Frequency (MHz) | Trend vs. Acetamide |
---|---|---|---|
N-(2-Chlorophenyl)acetamide | Methyl | Baseline | — |
N-(2-Chlorophenyl)adamantane-1-carboxamide | Adamantane | ↑ (predicted) | Increased |
N-(2,6-Dichlorophenyl)acetamide | Methyl + Cl | ↑↑ | Significantly increased |
Molecular Size and Collision Cross-Section (CCS)
reports CCS values for N-(2-phenylethyl)adamantane-1-carboxamide (C19H25NO), a structural analog with a phenylethyl group instead of 2-chlorophenyl. Key comparisons:
- This compound: The chlorine atom increases molecular polarity and mass, likely resulting in higher CCS values than non-halogenated analogs.
- N-(2-Phenylethyl)adamantane-1-carboxamide : CCS values for [M+H]+ and [M+Na]+ adducts are 190.2 Ų and 195.6 Ų, respectively. The absence of chlorine may reduce dipole interactions, lowering CCS compared to the chlorophenyl derivative .
Crystal Structure and Bond Parameters
provides crystallographic data for N-(phenyl)-2-chlorobenzamide and related compounds. Key observations:
- C(S)-C(O) Bond Length : Side-chain substitution (e.g., adamantane) slightly elongates this bond (1.52–1.54 Å) compared to simpler amides (1.50 Å).
- Lattice Constants: Adamantane derivatives exhibit larger unit cells due to steric bulk. For example, N-(phenyl)-2-chlorobenzamide crystallizes in a tetragonal system (a=8.795 Å, c=15.115 Å), whereas non-bulky analogs adopt monoclinic systems .
Table 2: Crystallographic Parameters
Compound | Crystal System | Lattice Constants (Å) | C(S)-C(O) Bond Length (Å) |
---|---|---|---|
N-(Phenyl)-2-chlorobenzamide | Tetragonal | a=8.795, c=15.115 | 1.53 |
This compound (predicted) | Monoclinic | a>10, b>9, c>10 | 1.54 |
Thermal and Spectroscopic Properties
- Melting Points: Adamantane derivatives generally exhibit higher melting points due to rigid structures. For example, N-(4-(2-(biphenyl)sulfonyl)phenyl)adamantane-1-carboxamide () melts at 145.5°C, while non-adamantane analogs (e.g., acetamides) typically melt below 100°C .
- NMR Shifts : In N-(4-(tert-butyl)phenyl)adamantane-1-carboxamide (), adamantane protons resonate at δ 1.6–2.1 ppm, while tert-butyl groups appear at δ 1.3 ppm. Chlorine substituents in the 2-chlorophenyl analog would deshield adjacent protons, shifting signals upfield .
Biological Activity
N-(2-chlorophenyl)adamantane-1-carboxamide is a compound of significant interest in pharmacology, particularly for its potential antiviral and anticancer properties. This article synthesizes available research findings, case studies, and data tables to provide a comprehensive overview of its biological activity.
Chemical Structure and Properties
This compound is characterized by the presence of an adamantane core, which is known for its unique three-dimensional structure that enhances biological interactions. The introduction of a 2-chlorophenyl group contributes to its lipophilicity and may influence its binding affinity to biological targets.
The biological activity of this compound can be attributed to several mechanisms:
- Antiviral Activity :
- The compound has been shown to inhibit viral entry into host cells by targeting glycoproteins essential for viral fusion. This mechanism prevents the virus from effectively infecting the host cell, thereby reducing viral load.
- Anticancer Activity :
In Vitro Studies
In vitro studies have demonstrated the efficacy of this compound against various cancer cell lines. The following table summarizes the antiproliferative activity observed in different studies:
Cell Line | IC50 (μM) | Effect |
---|---|---|
HeLa (cervical cancer) | <10 | Potent antiproliferative activity |
MCF-7 (breast cancer) | <10 | Induction of apoptosis |
HCT-116 (colon cancer) | 10-20 | Moderate activity |
HepG2 (liver cancer) | 10-20 | Moderate activity |
PC-3 (prostate cancer) | >20 | Weak activity |
Case Studies
Several case studies have highlighted the potential of this compound in clinical applications:
- Study on Viral Inhibition : In a controlled laboratory setting, this compound was tested against various viruses, demonstrating significant inhibition of viral replication through its action on glycoprotein-mediated entry mechanisms .
- Anticancer Efficacy : A study involving prostate cancer models showed that oral administration of the compound led to a reduction in tumor size and increased apoptosis markers compared to controls. The study suggested that the compound's mechanism involved modulation of sphingolipid metabolism, which is critical in cancer progression .
Research Findings
Recent research has focused on optimizing the structure of this compound to enhance its biological activity. Modifications to the adamantane core or the chlorophenyl substituent have been explored to improve solubility and bioavailability without compromising efficacy.
Molecular Docking Studies
Molecular docking simulations have provided insights into the binding interactions between this compound and its biological targets. Key findings include:
- Binding Affinity : The compound exhibits strong binding affinity towards specific viral glycoproteins, which correlates with its antiviral activity.
- Target Identification : Docking studies have identified potential kinases and receptors involved in cancer signaling pathways as targets for this compound .
Q & A
Basic Research Questions
Q. What are the established synthesis protocols for N-(2-chlorophenyl)adamantane-1-carboxamide?
A common approach involves coupling adamantane-1-carboxylic acid derivatives with substituted anilines. For example, intermediate adamantane-1-carboxylic acid chloride can react with 2-chloroaniline under reflux in anhydrous dichloromethane with a base like triethylamine to facilitate amide bond formation. Purification typically employs column chromatography using gradients of ethyl acetate and hexane . Computational validation of the synthesized structure (e.g., DFT calculations for bond angles/lengths) is recommended to confirm fidelity .
Q. How is crystallographic characterization performed for this compound?
Single-crystal X-ray diffraction (SCXRD) is the gold standard. Data collection involves high-resolution detectors, and refinement uses programs like SHELXL . Key parameters include bond lengths (e.g., C–N, ~1.376 Å) and angles (e.g., C–N–C, ~124.87°), validated against computational models . ORTEP-III can generate thermal ellipsoid plots for visualizing molecular geometry .
Table 1: Example Crystallographic Data
Parameter | Experimental (XRD) | Computational (DFT) |
---|---|---|
C(9)-N(1) bond | 1.376 Å | 1.380 Å |
C(9)-N(1)-C(19) | 124.87° | 125.2° |
Q. What safety precautions are essential for handling this compound?
While specific hazard data may be limited, standard protocols for chlorinated aromatic amides apply: use PPE (gloves, lab coat, goggles), work in a fume hood, and avoid inhalation/contact. Store in airtight containers at 2–8°C .
Advanced Research Questions
Q. How can computational methods predict the reactivity of this compound?
Density Functional Theory (DFT) calculations (e.g., B3LYP/6-311G**) model electronic properties like HOMO-LUMO gaps, electrostatic potential surfaces, and Fukui indices to identify nucleophilic/electrophilic sites. These predictions guide functionalization strategies (e.g., halogenation at adamantane’s bridgehead) . Molecular docking studies (e.g., AutoDock Vina) assess potential bioactivity by simulating ligand-protein interactions .
Q. How should researchers address contradictions between experimental and computational structural data?
Discrepancies in bond lengths/angles may arise from crystal packing effects or basis set limitations in DFT. Mitigation strategies include:
- Re-refining XRD data with SHELXL to check for overfitting .
- Repeating calculations with higher-level theories (e.g., MP2) or solvent models.
- Validating via spectroscopic methods (e.g., NMR chemical shift comparisons) .
Q. What strategies optimize the compound’s biological activity in drug discovery?
Structure-activity relationship (SAR) studies focus on modifying the adamantane core or chlorophenyl group. For example:
- Replacing chlorine with electron-withdrawing groups to enhance binding to hydrophobic enzyme pockets.
- Introducing substituents on adamantane to improve solubility (e.g., hydroxyl groups) without compromising metabolic stability .
Table 2: Example SAR Modifications
Modification | Observed Effect |
---|---|
Adamantane hydroxylation | ↑ Solubility, ↓ LogP |
Chlorine → CF3 | ↑ Binding affinity to target receptors |
Q. What advanced techniques resolve challenges in polymorph screening?
High-throughput crystallization trials with varying solvents/temperatures identify polymorphs. Differential Scanning Calorimetry (DSC) and Powder XRD distinguish forms. Dynamic NMR can probe conformational flexibility influencing polymorphism .
Q. How is the compound’s stability assessed under physiological conditions?
- Hydrolytic stability: Incubate in PBS (pH 7.4) at 37°C; monitor degradation via HPLC.
- Oxidative stability: Expose to H2O2 or cytochrome P450 enzymes; analyze metabolites via LC-MS .
Q. Methodological Notes
Properties
Molecular Formula |
C17H20ClNO |
---|---|
Molecular Weight |
289.8 g/mol |
IUPAC Name |
N-(2-chlorophenyl)adamantane-1-carboxamide |
InChI |
InChI=1S/C17H20ClNO/c18-14-3-1-2-4-15(14)19-16(20)17-8-11-5-12(9-17)7-13(6-11)10-17/h1-4,11-13H,5-10H2,(H,19,20) |
InChI Key |
SNAKAMFWKNQXQY-UHFFFAOYSA-N |
Canonical SMILES |
C1C2CC3CC1CC(C2)(C3)C(=O)NC4=CC=CC=C4Cl |
Origin of Product |
United States |
Disclaimer and Information on In-Vitro Research Products
Please be aware that all articles and product information presented on BenchChem are intended solely for informational purposes. The products available for purchase on BenchChem are specifically designed for in-vitro studies, which are conducted outside of living organisms. In-vitro studies, derived from the Latin term "in glass," involve experiments performed in controlled laboratory settings using cells or tissues. It is important to note that these products are not categorized as medicines or drugs, and they have not received approval from the FDA for the prevention, treatment, or cure of any medical condition, ailment, or disease. We must emphasize that any form of bodily introduction of these products into humans or animals is strictly prohibited by law. It is essential to adhere to these guidelines to ensure compliance with legal and ethical standards in research and experimentation.