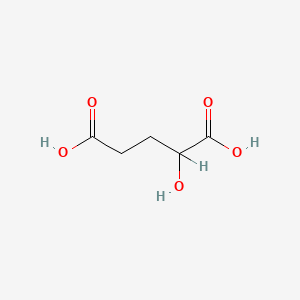
2-Hydroxyglutaric acid
Overview
Description
2-Hydroxyglutaric acid (2-HG) is a metabolite structurally similar to α-ketoglutarate (α-KG), a crucial intermediate of the tricarboxylic acid (TCA) cycle. It is generated by the reduction of the ketone group of α-KG to a hydroxyl group. 2-HG has gained significant attention due to its role in various pathophysiological conditions, including 2-hydroxyglutaric aciduria (2HGA), tumors with mutant isocitrate dehydrogenase 1/2 (IDH1/2mt), and clear cell renal cell carcinoma (ccRCC) (Xin Du & Hai Hu, 2021).
Scientific Research Applications
1. Role in Metabolic Encephalopathy
2-Hydroxyglutaric aciduria is a rare metabolic encephalopathy that manifests as a subcortical leukoencephalopathy, visible in MRI scans. The disease involves abnormal accumulation of L-2-hydroxyglutaric acid in body fluids and is associated with the development of malignant brain tumors, suggesting a potential role in brain tumorigenesis (Moroni et al., 2004).
2. Genetic and Biochemical Insights
Research has identified mutations in genes encoding enzymes involved in the metabolism of L-2-hydroxyglutaric acid. These mutations lead to elevated concentrations of L-2-hydroxyglutaric acid in urine, plasma, and cerebrospinal fluid, which are characteristic of L-2-hydroxyglutaric aciduria. This disorder is due to a toxic effect of L-2-hydroxyglutaric acid on the central nervous system (Rzem et al., 2004).
3. Oncogenic Mechanism and Immune Cell Fate
2-Hydroxyglutarate plays a significant role in the pathophysiology of 2-hydroxyglutaric aciduria, certain tumors, and clear cell renal cell carcinoma. It is considered an oncometabolite and is involved in the fate decision of immune cells, suggesting its broader implications beyond tumorigenesis (Du & Hu, 2021).
4. Diagnostic Techniques and Clinical Studies
Advanced diagnostic techniques like field asymmetric waveform ion mobility spectrometry/mass spectrometry have been developed for rapid discrimination of 2-hydroxyglutaric acid, important for clinical analysis and understanding its role in various diseases (Fukui et al., 2021). Clinical and neuroradiological findings in patients with L-2-hydroxyglutaric aciduria provide insights into its neurological impacts and progression (Moroni et al., 2000).
5. Molecular Defects in L-2-Hydroxyglutaric Aciduria
Studies have identified compound heterozygous mutations in genes related to L-2-hydroxyglutaric aciduria, revealing insights into the molecular basis and metabolic pathways affected by these mutations (Zhang et al., 2018).
6. Neuropathological Correlations
Neuropathological studies provide a deeper understanding of the effects of L-2-hydroxyglutaric aciduria on brain development and function. These include investigations into brain atrophy, neuronal loss, and the unique characteristics of brain lesions associated with the disorder (Chen et al., 1996).
7. Neuroimaging and Spectroscopy Findings
Magnetic resonance imaging (MRI) and spectroscopy have been crucial in diagnosing and understanding the progression of L-2-hydroxyglutaric aciduria. They reveal specific patterns in brain structure and function, aiding in the clinical management of the disorder (Aydin et al., 2003).
Mechanism of Action
Target of Action
2-Hydroxyglutaric acid (2-HG) primarily targets two enzymes in humans, D2HGDH and L2HGDH, which convert 2-HG to α-ketoglutaric acid . It also targets isocitrate dehydrogenase (IDH1 and IDH2), which frequently mutate in glioma and AML .
Mode of Action
2-HG is structurally similar to α-ketoglutarate (α-KG), an intermediate product of the tricarboxylic acid (TCA) cycle . It can be generated by reducing the ketone group of α-KG to a hydroxyl group . In humans, the compound is formed by a hydroxyacid-oxoacid transhydrogenase . The compound can be converted to α-ketoglutaric acid through the action of a 2-hydroxyglutarate dehydrogenase .
Biochemical Pathways
2-HG plays a significant role in the pathophysiology of 2-hydroxyglutaric aciduria (2HGA), tumors harboring mutant isocitrate dehydrogenase 1/2 (IDH1/2mt), and in clear cell renal cell carcinoma (ccRCC) . It is taken as an oncometabolite, raising much attention on its oncogenic mechanism .
Pharmacokinetics
It is known that 2-hg accumulates in the context of hypoxia or acidic ph .
Result of Action
D-2-hydroxyglutarate accumulates to very high concentrations which inhibits the function of enzymes that are dependent on alpha-ketoglutarate, including histone lysine demethylases . This leads to a hypermethylated state of DNA and histones, which results in different gene expression that can activate oncogenes and inactivate tumor-suppressor genes .
Safety and Hazards
Future Directions
Uncovering further molecular metabolism details specific for given cancer cell types and sequence-specific epigenetic alternations will lead to the design of diagnostic approaches, not only for predicting patients’ prognosis or uncovering metastases and tumor remissions but also for early diagnostics .
properties
IUPAC Name |
2-hydroxypentanedioic acid | |
---|---|---|
Source | PubChem | |
URL | https://pubchem.ncbi.nlm.nih.gov | |
Description | Data deposited in or computed by PubChem | |
InChI |
InChI=1S/C5H8O5/c6-3(5(9)10)1-2-4(7)8/h3,6H,1-2H2,(H,7,8)(H,9,10) | |
Source | PubChem | |
URL | https://pubchem.ncbi.nlm.nih.gov | |
Description | Data deposited in or computed by PubChem | |
InChI Key |
HWXBTNAVRSUOJR-UHFFFAOYSA-N | |
Source | PubChem | |
URL | https://pubchem.ncbi.nlm.nih.gov | |
Description | Data deposited in or computed by PubChem | |
Canonical SMILES |
C(CC(=O)O)C(C(=O)O)O | |
Source | PubChem | |
URL | https://pubchem.ncbi.nlm.nih.gov | |
Description | Data deposited in or computed by PubChem | |
Molecular Formula |
C5H8O5 | |
Source | PubChem | |
URL | https://pubchem.ncbi.nlm.nih.gov | |
Description | Data deposited in or computed by PubChem | |
Related CAS |
40951-21-1 (di-hydrochloride salt) | |
Record name | alpha-Hydroxyglutarate | |
Source | ChemIDplus | |
URL | https://pubchem.ncbi.nlm.nih.gov/substance/?source=chemidplus&sourceid=0002889318 | |
Description | ChemIDplus is a free, web search system that provides access to the structure and nomenclature authority files used for the identification of chemical substances cited in National Library of Medicine (NLM) databases, including the TOXNET system. | |
DSSTOX Substance ID |
DTXSID40864360 | |
Record name | 2-Hydroxypentanedioic acid | |
Source | EPA DSSTox | |
URL | https://comptox.epa.gov/dashboard/DTXSID40864360 | |
Description | DSSTox provides a high quality public chemistry resource for supporting improved predictive toxicology. | |
Molecular Weight |
148.11 g/mol | |
Source | PubChem | |
URL | https://pubchem.ncbi.nlm.nih.gov | |
Description | Data deposited in or computed by PubChem | |
Physical Description |
Solid | |
Record name | 2-Hydroxyglutarate | |
Source | Human Metabolome Database (HMDB) | |
URL | http://www.hmdb.ca/metabolites/HMDB0059655 | |
Description | The Human Metabolome Database (HMDB) is a freely available electronic database containing detailed information about small molecule metabolites found in the human body. | |
Explanation | HMDB is offered to the public as a freely available resource. Use and re-distribution of the data, in whole or in part, for commercial purposes requires explicit permission of the authors and explicit acknowledgment of the source material (HMDB) and the original publication (see the HMDB citing page). We ask that users who download significant portions of the database cite the HMDB paper in any resulting publications. | |
CAS RN |
2889-31-8 | |
Record name | (±)-2-Hydroxyglutaric acid | |
Source | CAS Common Chemistry | |
URL | https://commonchemistry.cas.org/detail?cas_rn=2889-31-8 | |
Description | CAS Common Chemistry is an open community resource for accessing chemical information. Nearly 500,000 chemical substances from CAS REGISTRY cover areas of community interest, including common and frequently regulated chemicals, and those relevant to high school and undergraduate chemistry classes. This chemical information, curated by our expert scientists, is provided in alignment with our mission as a division of the American Chemical Society. | |
Explanation | The data from CAS Common Chemistry is provided under a CC-BY-NC 4.0 license, unless otherwise stated. | |
Record name | alpha-Hydroxyglutarate | |
Source | ChemIDplus | |
URL | https://pubchem.ncbi.nlm.nih.gov/substance/?source=chemidplus&sourceid=0002889318 | |
Description | ChemIDplus is a free, web search system that provides access to the structure and nomenclature authority files used for the identification of chemical substances cited in National Library of Medicine (NLM) databases, including the TOXNET system. | |
Record name | 2-Hydroxypentanedioic acid | |
Source | EPA DSSTox | |
URL | https://comptox.epa.gov/dashboard/DTXSID40864360 | |
Description | DSSTox provides a high quality public chemistry resource for supporting improved predictive toxicology. | |
Record name | 2-HYDROXYGLUTARIC ACID, (±)- | |
Source | FDA Global Substance Registration System (GSRS) | |
URL | https://gsrs.ncats.nih.gov/ginas/app/beta/substances/RS4M3UYS95 | |
Description | The FDA Global Substance Registration System (GSRS) enables the efficient and accurate exchange of information on what substances are in regulated products. Instead of relying on names, which vary across regulatory domains, countries, and regions, the GSRS knowledge base makes it possible for substances to be defined by standardized, scientific descriptions. | |
Explanation | Unless otherwise noted, the contents of the FDA website (www.fda.gov), both text and graphics, are not copyrighted. They are in the public domain and may be republished, reprinted and otherwise used freely by anyone without the need to obtain permission from FDA. Credit to the U.S. Food and Drug Administration as the source is appreciated but not required. | |
Record name | 2-Hydroxyglutarate | |
Source | Human Metabolome Database (HMDB) | |
URL | http://www.hmdb.ca/metabolites/HMDB0059655 | |
Description | The Human Metabolome Database (HMDB) is a freely available electronic database containing detailed information about small molecule metabolites found in the human body. | |
Explanation | HMDB is offered to the public as a freely available resource. Use and re-distribution of the data, in whole or in part, for commercial purposes requires explicit permission of the authors and explicit acknowledgment of the source material (HMDB) and the original publication (see the HMDB citing page). We ask that users who download significant portions of the database cite the HMDB paper in any resulting publications. | |
Retrosynthesis Analysis
AI-Powered Synthesis Planning: Our tool employs the Template_relevance Pistachio, Template_relevance Bkms_metabolic, Template_relevance Pistachio_ringbreaker, Template_relevance Reaxys, Template_relevance Reaxys_biocatalysis model, leveraging a vast database of chemical reactions to predict feasible synthetic routes.
One-Step Synthesis Focus: Specifically designed for one-step synthesis, it provides concise and direct routes for your target compounds, streamlining the synthesis process.
Accurate Predictions: Utilizing the extensive PISTACHIO, BKMS_METABOLIC, PISTACHIO_RINGBREAKER, REAXYS, REAXYS_BIOCATALYSIS database, our tool offers high-accuracy predictions, reflecting the latest in chemical research and data.
Strategy Settings
Precursor scoring | Relevance Heuristic |
---|---|
Min. plausibility | 0.01 |
Model | Template_relevance |
Template Set | Pistachio/Bkms_metabolic/Pistachio_ringbreaker/Reaxys/Reaxys_biocatalysis |
Top-N result to add to graph | 6 |
Feasible Synthetic Routes
Q & A
Q1: What are the two main types of 2-hydroxyglutaric aciduria, and how are they differentiated?
A1: The two main types are D-2-hydroxyglutaric aciduria and L-2-hydroxyglutaric aciduria. They are differentiated based on which enantiomer of 2-hydroxyglutaric acid is found in excess in the urine of patients. [] Accurate diagnosis relies on determining if the excess enantiomer is D-2-hydroxyglutaric acid or L-2-hydroxyglutaric acid. [, , ]
Q2: What is the biochemical hallmark of L-2-hydroxyglutaric aciduria?
A2: The biochemical hallmark is the accumulation of L-2-hydroxyglutaric acid in cerebrospinal fluid, plasma, and urine. []
Q3: What are the typical clinical manifestations of L-2-hydroxyglutaric aciduria?
A3: Common symptoms include mental retardation, seizures, and ataxia. [] Other symptoms include psychomotor regression starting in infancy, mild choreodystonia, pyramidal signs, and epilepsy. []
Q4: What are the characteristic neuroimaging findings in L-2-hydroxyglutaric aciduria?
A4: Brain MRI typically reveals bilateral symmetrical abnormalities in subcortical white matter, basal ganglia, and dentate nucleus. [] Confluent subcortical white matter lesions and minimal basal ganglia abnormalities are also observed. []
Q5: What is the genetic basis of L-2-hydroxyglutaric aciduria?
A6: L-2-hydroxyglutaric aciduria is caused by mutations in the L2HGDH gene located on chromosome 14q22.1. [] This gene encodes L-2-hydroxyglutarate dehydrogenase, an enzyme responsible for metabolizing L-2-hydroxyglutaric acid to α-ketoglutarate. []
Q6: What is the function of the enzyme L-2-hydroxyglutarate dehydrogenase?
A7: This enzyme catalyzes the oxidation of L-2-hydroxyglutarate to α-ketoglutarate. [] It is a flavin adenine dinucleotide (FAD)-dependent enzyme primarily found in the liver and kidneys but also present in lower levels in the heart, brain, and other tissues. []
Q7: What are the potential therapeutic targets for L-2-hydroxyglutaric aciduria?
A8: While no cure exists, some patients may benefit from high doses of riboflavin (vitamin B2) and levocarnitine. [] These treatments may improve behavioral symptoms and potentially slow disease progression. []
Q8: Is there a link between L-2-hydroxyglutaric aciduria and brain tumors?
A9: Yes, an increased incidence of brain tumors has been observed in some patients with L-2-hydroxyglutaric aciduria. [, ] Further research is needed to fully understand the relationship between elevated L-2-hydroxyglutaric acid levels and tumorigenesis.
Q9: What is the significance of the c.169G>A mutation in the L2HGDH gene?
A10: This mutation has been found in L-2-hydroxyglutaric aciduria patients of different ethnicities, including Italian, Portuguese, and Arab populations. [] While the reoccurrence of the same mutation in diverse populations is rare, the c.169G>A mutation appears to have arisen independently in Arab patients. []
Q10: What animal models are available for studying L-2-hydroxyglutaric aciduria?
A11: Staffordshire Bull Terriers have been identified as a spontaneous model for L-2-hydroxyglutaric aciduria. [, ] These dogs exhibit similar clinical signs to humans, including seizures, ataxia, dementia, and tremors, making them valuable for investigating the disease mechanism and potential therapies. [, ]
Q11: How is D-2-hydroxyglutaric aciduria diagnosed?
A12: Similar to L-2-hydroxyglutaric aciduria, diagnosis relies on detecting elevated D-2-hydroxyglutaric acid in body fluids using techniques like gas chromatography-mass spectrometry. []
Q12: What are the characteristic neuroimaging findings in D-2-hydroxyglutaric aciduria?
A13: Typical MRI findings include delayed cerebral maturation, ventricular abnormalities, and sub-ependymal cysts, particularly in the first few months of life. []
Q13: What is the potential link between D-2-hydroxyglutaric aciduria and glutaric aciduria type 1?
A14: While the exact relationship remains unclear, a study reported siblings diagnosed with both conditions. [] The sibling with glutaric aciduria type 1 also exhibited a slight increase in D-2-hydroxyglutaric acid excretion. [] Further research is needed to determine any pathophysiological link between these disorders.
Q14: What is the significance of this compound in cancer research?
A15: this compound, particularly its D-enantiomer, is considered an oncometabolite. [, ] Its accumulation, often due to mutations in isocitrate dehydrogenase enzymes (IDH1 and IDH2), is linked to various cancers. [, ]
Q15: How do mutant IDH1 inhibitors exert their anti-cancer effects?
A16: These inhibitors selectively target mutant IDH1, preventing the conversion of α-ketoglutarate to D-2-hydroxyglutaric acid. [] By reducing D-2-hydroxyglutaric acid levels, these inhibitors aim to reverse the oncometabolite's effects on cell proliferation and differentiation. []
Q16: How does the mechanism of action differ between wild-type and mutant IDH1 inhibitors?
A17: Mutant-selective IDH1 inhibitors typically bind to an allosteric site on the enzyme, competing with magnesium ions essential for catalytic activity. [] This binding disrupts the metal-binding network, specifically targeting the mutant enzyme while leaving the wild-type IDH1 relatively unaffected. []
Q17: What are the analytical challenges associated with this compound analysis?
A18: One challenge is the need for chiral separation to differentiate between D- and L-enantiomers, as they have distinct biological properties and clinical significance. [, , ]
Q18: What methods are commonly used for the chiral separation and analysis of this compound?
A18: Several techniques are employed, including:
- Chiral derivatization combined with gas chromatography and mass spectrometry (GC/MS): This involves reacting this compound with a chiral reagent, followed by separation and detection using GC/MS. [, ]
- Chiral liquid chromatography tandem mass spectrometry (LC-MS/MS): This technique utilizes a chiral stationary phase in the chromatography column to separate the enantiomers, which are then detected by mass spectrometry. [, , ]
- Capillary electrophoresis with capacitively coupled contactless conductivity detection: This method separates the enantiomers based on their differential migration in an electric field using a chiral selector, followed by detection using a contactless conductivity detector. []
Q19: What are the advantages of using chiral derivatization in this compound analysis?
A20: This method enhances the sensitivity and selectivity of analysis, enabling the detection of this compound enantiomers at low concentrations in complex biological matrices. []
Q20: What are the limitations of conventional methods for this compound enantiomer separation?
A21: Traditional techniques often require multiple analytical instruments, specialized expertise, and long run times, limiting their practicality for high-throughput clinical analysis. []
Q21: What novel approaches are being explored for rapid chiral discrimination of this compound?
A22: One promising approach combines chiral derivatization with field asymmetric waveform ion mobility spectrometry/mass spectrometry (FAIMS/MS). [] This method utilizes a chiral derivatizing reagent and the differential mobility of enantiomer ions in an electric field for rapid separation (less than 1 second) and detection. []
Q22: What is the significance of detecting D-2-hydroxyglutaric acid in cancer patients?
A23: Elevated D-2-hydroxyglutaric acid in tumors can indicate the presence of specific pathogenic mutations, particularly in IDH1 and IDH2 genes. [] Monitoring D-2-hydroxyglutaric acid levels could potentially serve as a biomarker for diagnosing these mutations and monitoring treatment response. []
Q23: What is the role of this compound in fungal metabolism?
A24: In Aspergillus glaucus, this compound is an intermediate in the metabolism of propionate. [] The fungus incorporates glyoxylic acid into this compound, which is then further metabolized, likely to lactate and acetate. [, ]
Q24: What is the potential connection between the serA gene and human 2-hydroxyglutaric aciduria?
A25: The serA gene in Escherichia coli encodes 3-phosphoglycerate dehydrogenase, an enzyme involved in L-serine biosynthesis. [] Interestingly, this enzyme also exhibits an alpha-ketoglutarate reductase activity, catalyzing the formation of both D- and L-2-hydroxyglutaric acid. [] This finding suggests that a mutation in the human homolog of the serA gene could potentially contribute to D- and L-2-hydroxyglutaric aciduria. []
Disclaimer and Information on In-Vitro Research Products
Please be aware that all articles and product information presented on BenchChem are intended solely for informational purposes. The products available for purchase on BenchChem are specifically designed for in-vitro studies, which are conducted outside of living organisms. In-vitro studies, derived from the Latin term "in glass," involve experiments performed in controlled laboratory settings using cells or tissues. It is important to note that these products are not categorized as medicines or drugs, and they have not received approval from the FDA for the prevention, treatment, or cure of any medical condition, ailment, or disease. We must emphasize that any form of bodily introduction of these products into humans or animals is strictly prohibited by law. It is essential to adhere to these guidelines to ensure compliance with legal and ethical standards in research and experimentation.