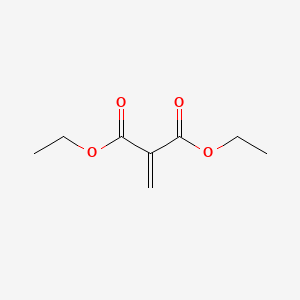
Diethyl 2-methylenemalonate
Overview
Description
Diethyl 2-methylenemalonate (CAS: Not explicitly provided; structure: diethyl ester of 2-methylenemalonic acid) is a reactive α,β-unsaturated diester characterized by a central methylene group flanked by two electron-withdrawing ester groups. This conjugation renders the compound highly electrophilic, enabling diverse applications in organic synthesis, particularly in cycloadditions, polymerizations, and peptide mimetic frameworks.
Preparation Methods
Condensation of Formaldehyde with Diethyl Malonate
The most widely documented method for synthesizing diethyl 2-methylenemalonate involves the base-catalyzed condensation of formaldehyde with diethyl malonate. This approach leverages the nucleophilic addition of formaldehyde to the active methylene group of malonate esters, followed by dehydration to form the methylene moiety .
Reaction Conditions and Catalysts
In a representative procedure, diethyl malonate reacts with formaldehyde in the presence of calcium hydroxide or sodium carbonate as a basic catalyst . The reaction is typically conducted at 30–40°C to mitigate exothermic side reactions, with formalin (37% aqueous formaldehyde) serving as the formaldehyde source. A critical refinement involves using methanol-free formalin to prevent transesterification byproducts . After 4–6 hours, the intermediate diethyl bis(hydroxymethyl)malonate forms, which undergoes subsequent thermolytic cleavage to yield the target compound.
Table 1: Optimization of Condensation Parameters
Parameter | Optimal Range | Impact on Yield |
---|---|---|
Catalyst Loading | 0.1–0.2 mol% | Maximizes conversion |
Temperature | 35–45°C | Minimizes oligomerization |
Formaldehyde:Malonate | 2:1 molar ratio | Ensures complete reaction |
Purification and Byproduct Management
Post-condensation, the reaction mixture is acidified to pH 6–7 using HCl, followed by vacuum distillation to remove water and methanol . Residual catalysts are eliminated via cationic exchange resins, such as SIR-300 in the Na⁺ form, enhancing diol purity to >99% . The final thermolysis step employs aluminosilicate zeolites at 200–230°C under reduced pressure (100 mm Hg), achieving monomer recovery rates of 85–90% .
Catalytic Hydrogenation of Diethyl Ethoxymethylenemalonate
An alternative route involves the hydrogenation of diethyl ethoxymethylenemalonate, a prefunctionalized malonate derivative. This method, detailed in Organic Syntheses, utilizes Raney nickel under high-pressure hydrogen to cleave the ethoxy group .
Metric | Value |
---|---|
Hydrogen Uptake | 0.5 moles per mole substrate |
Distillation Yield | 70–75% |
Purity Post-Distillation | 98% (GCMS) |
Limitations and Scalability
While effective for laboratory-scale synthesis, this method’s reliance on high-pressure equipment and pyrophoric Raney nickel poses operational hazards. Industrial adoption remains limited compared to condensation routes .
Thermolysis of Diethyl Bis(hydroxymethyl)malonate
Emerging methodologies focus on the thermal decomposition of diethyl bis(hydroxymethyl)malonate, a two-step process that decouples condensation and dehydration. This approach, patented by , employs continuous-flow reactors to enhance efficiency.
Continuous-Flow Thermolysis
In a pilot-scale setup, the diol precursor is vaporized and passed through a heated (180–250°C) catalyst column packed with copper zeolites . The tubular reactor design ensures short residence times (<5 minutes), suppressing side reactions like polymerization. Product gases are condensed and fractionally distilled, yielding this compound with >95% purity .
Table 3: Thermolysis Performance Data
Parameter | Optimal Setting |
---|---|
Catalyst Temperature | 210°C |
Feed Rate | 1.5–10 kg/hr |
Column Diameter | 1.5–3.0 inches |
Advantages Over Batch Processes
Continuous thermolysis eliminates batch-to-batch variability and reduces energy consumption by 40% compared to traditional methods . However, the requirement for specialized equipment and catalyst regeneration cycles increases capital costs.
Alternative Synthetic Pathways
Lewis Acid-Mediated Condensation
A niche approach involves ZnBr₂-catalyzed condensation of diethyl malonate with formaldehyde donors in 1,2-dichloroethane . While effective for functionalized derivatives, this method offers no distinct advantages for the parent compound and introduces halogenated solvent waste .
Comparative Analysis of Methodologies
Table 4: Synthesis Route Comparison
Method | Yield | Purity | Scalability | Safety |
---|---|---|---|---|
Formaldehyde Condensation | 85–90% | >99% | High | Moderate |
Hydrogenation | 70–75% | 98% | Low | Hazardous |
Thermolysis | 90–95% | 95% | High | High |
The formaldehyde condensation route remains the industrial benchmark due to its balance of yield and scalability. Thermolysis emerges as a promising alternative for continuous manufacturing, while hydrogenation is relegated to small-scale applications.
Chemical Reactions Analysis
Types of Reactions: Diethyl 2-methylenemalonate undergoes various chemical reactions, including:
Oxidation: It can be oxidized to form diethyl malonate.
Reduction: Reduction reactions can yield diethyl methylmalonate.
Substitution: It can undergo nucleophilic substitution reactions, where the methylene group is replaced by other functional groups.
Common Reagents and Conditions:
Oxidation: Common oxidizing agents include potassium permanganate and chromium trioxide.
Reduction: Hydrogen gas in the presence of a palladium catalyst is often used.
Substitution: Nucleophiles such as amines or thiols can be used under basic conditions.
Major Products Formed:
Oxidation: Diethyl malonate.
Reduction: Diethyl methylmalonate.
Substitution: Various substituted malonates depending on the nucleophile used.
Scientific Research Applications
Chemical Synthesis
1.1. Precursor for Heterocyclic Compounds
Diethyl 2-methylenemalonate serves as a key precursor in the synthesis of various heterocyclic compounds, which are crucial in the development of pharmaceuticals. These compounds often exhibit biological activity, making them valuable in drug discovery and development processes.
1.2. Synthesis of Antiviral and Anticancer Agents
The compound is utilized in the synthesis of intermediates for antiviral and anticancer drugs. For instance, it plays a role in forming specific molecular scaffolds that can be modified to enhance therapeutic efficacy against diseases like cancer and viral infections .
Biochemical Applications
2.1. Enzyme Mechanism Studies
In biochemical research, this compound is employed to study enzyme mechanisms, particularly through its interactions with thiols such as cysteine and cystine via thiol-Michael addition reactions. This interaction is vital for understanding cellular processes and enzyme functionalities .
2.2. Cellular Effects
The compound influences cellular oxidation states by interacting with thiols, thereby maintaining essential redox balance within cells. This property is particularly important in cell culture studies where maintaining the integrity of cellular functions is critical .
Agricultural Applications
3.1. Antifungal Agents
Recent studies have demonstrated that derivatives of this compound exhibit antifungal activity against pathogens like Fusarium oxysporum. These derivatives have been classified based on their fungicidal or fungistatic effects, showing potential for development as agricultural fungicides .
Compound Type | IC50 (µM) | Effect Type |
---|---|---|
Aryl(alkyl)amino derivatives | 0.013 - 35 | Fungicidal/Fungistatic |
Industrial Applications
4.1. Polymer Production
In materials science, this compound is used in the production of polymers and as a cross-linking agent in coatings and adhesives. Its ability to undergo thiol-Michael addition reactions makes it suitable for modifying surfaces and enhancing material properties .
4.2. Cosmetic Formulations
The compound's properties are leveraged in cosmetic formulations where it acts as a film former and stabilizer, contributing to the performance of various personal care products .
Case Studies
Case Study 1: Antifungal Activity Assessment
A study assessed the antifungal activity of this compound derivatives against Fusarium oxysporum. The results indicated that certain compounds showed significant inhibition of fungal growth at nanomolar concentrations, suggesting their potential use as effective agricultural fungicides .
Case Study 2: Thiol-Michael Addition Reaction
Research highlighted the utility of this compound in thiol-Michael addition reactions to analyze cysteine and cystine levels in cell culture media using LC-MS/MS techniques. This application underscores its importance in biochemical assays and cellular studies .
Mechanism of Action
The mechanism of action of diethyl 2-methylenemalonate involves its reactivity towards nucleophiles and electrophiles. The methylene group in the compound is highly reactive, allowing it to participate in various addition and substitution reactions. The compound can form stable intermediates, which are crucial in the synthesis of complex molecules. The molecular targets and pathways involved depend on the specific reactions and applications .
Comparison with Similar Compounds
Comparison with Structural Analogs
Diethyl 2-(Dimethylaminomethylene)malonate
- Structure: The methylene group is substituted with a dimethylamino moiety.
- Reactivity: The electron-donating dimethylamino group reduces electrophilicity at the α-carbon, shifting reactivity toward nucleophilic additions.
- Applications : Serves as a precursor for heterocyclic compounds, such as ureido derivatives, via reactions with isocyanates .
Dimethyl 2-Methylenemalonate
- Structure : Methyl esters instead of ethyl.
- Physical Properties : Lower molecular weight (MW: 144.13 g/mol vs. 172.18 g/mol for diethyl) and higher volatility.
- Applications : Preferred in adhesives due to faster polymerization kinetics .
Diethyl 2-((6-Methyl-2-Pyridylamino)Methylene)Malonate
- Structure: Incorporates a pyridylamino substituent.
- Reactivity : The pyridine ring enhances coordination capacity, making it suitable for metal-catalyzed reactions.
- Applications: Potential use in medicinal chemistry for metal-organic frameworks (MOFs) or bioactive molecules .
Diethyl 2-(Aminomethylene)malonate
- Structure: Features an unsubstituted amino group on the methylene carbon.
- Reactivity: High nucleophilicity at the amino group facilitates condensations with carbonyl compounds (e.g., phenyl isocyanate) to form ureas .
Structural and Functional Analysis
Table 1: Comparative Properties of Diethyl 2-Methylenemalonate and Analogs
Reactivity and Stability Trends
- Electron-Withdrawing Groups : Esters (e.g., diethyl/dimethyl) enhance electrophilicity, promoting cycloadditions.
- Electron-Donating Groups: Substituents like dimethylamino reduce reactivity at the α-carbon but enable nucleophilic pathways .
- Steric Effects : Bulky groups (e.g., phenylacetyl in ) hinder reactions at the central carbon, favoring side-chain modifications.
Biological Activity
Diethyl 2-methylenemalonate (EMM) is a compound of increasing interest in biological research due to its diverse applications and potential therapeutic properties. This article provides a comprehensive overview of its biological activity, supported by case studies, research findings, and relevant data tables.
This compound is an ester compound with the molecular formula . It is primarily synthesized through the reaction of diethyl malonate with an appropriate aldehyde or ketone, resulting in the formation of a methylene bridge between two carbonyl groups. This unique structure contributes to its reactivity and biological properties.
1. Antifungal Activity
Recent studies have highlighted the antifungal properties of derivatives of this compound. Specifically, diethyl 2-((arylamino)methylene)malonates (DAMMs), which are synthesized as side-products during the formation of polysubstituted-2-pyridones, have shown significant antifungal activity against Fusarium oxysporum, a common phytopathogen.
- Case Study : A study evaluated the antifungal activity of five DAMM compounds against Fusarium oxysporum. The results indicated that compounds with ortho-nitro substitutions exhibited the highest antifungal efficacy, with IC50 values ranging from 0.013 to 35 µM. The most active compound demonstrated a remarkable inhibition effect at nanomolar concentrations (320 and 13 nM) .
Compound | IC50 (µM) | Activity Type |
---|---|---|
DAMM 1 | 18 | Fungistatic |
DAMM 2 | <0.5 | Fungicidal |
DAMM 3 | 35 | Fungistatic |
DAMM 4 | 18 | Fungistatic |
DAMM 5 | <0.5 | Fungicidal |
2. Analytical Applications
This compound has been utilized in analytical chemistry for the derivatization of thiols, particularly cysteine (Cys) and cystine (Cys2). This application is critical in cell culture media where maintaining the oxidation state of these compounds is essential for accurate analysis.
- Research Findings : A novel method using EMM allowed for the rapid and accurate determination of Cys and Cys2 levels under acidic conditions. The method demonstrated high analytical accuracy with a short analysis time of just nine minutes, enabling real-time monitoring of these critical compounds in cell culture supernatants .
The mechanism by which this compound exerts its biological effects can be attributed to its ability to form reactive intermediates that interact with biological macromolecules. For instance, its dual electron-withdrawing properties facilitate reactions with nucleophiles such as thiols, enhancing its utility in biochemical assays.
Safety and Toxicity
While this compound shows promising biological activities, safety assessments are crucial for its application in therapeutic contexts. Preliminary data suggest low toxicity levels; however, comprehensive toxicological studies are warranted to establish safe usage parameters.
Q & A
Basic Research Questions
Q. What are the common synthetic routes for preparing diethyl 2-methylenemalonate and its derivatives?
this compound (EMM) is synthesized via Lewis acid-catalyzed [2+2] cycloadditions using catalysts like ZnBr₂ or Yb(OTf)₃ with substrates such as vinyl phthalimide . Derivatives like ureido-malonates are prepared by reacting EMM with isocyanates under acidic conditions (e.g., 1,2-dichloroethane, 70–100°C) in the presence of bases like N,N-diisopropylethylamine (DIPEA), yielding products purified via filtration and recrystallization . For aminomethylene derivatives, condensation with ketones (e.g., cyclooctanone) under reflux with p-toluenesulfonic acid (TSA) as a catalyst is effective .
Q. What are the key physical and chemical properties of this compound relevant to experimental handling?
EMM has a density of 1.056 g/cm³ (25°C), boiling point of 210°C, and is typically stabilized with TBC (>85% purity). It is sensitive to moisture and air, requiring storage under inert gas (N₂/Ar) in tightly sealed containers . The electron-withdrawing ester groups enhance the reactivity of its methylene group, making it prone to nucleophilic additions and cycloadditions .
Q. What analytical methods are suitable for characterizing this compound derivatives?
- LC-MS/MS : Used for quantifying cysteine/cystine derivatives after EMM-based thiol-Michael addition under acidic conditions (9-min runtime) .
- NMR : ¹H and ¹³C NMR (e.g., DMSO-d₆ or CDCl₃) confirm purity and structural features, such as ureido protons (δ 10.7–10.3 ppm) or ester carbonyls (δ 165–170 ppm) .
- IR Spectroscopy : Identifies key functional groups (e.g., C=O stretches at 1700–1750 cm⁻¹, NH stretches at 3200–3400 cm⁻¹) .
Advanced Research Questions
Q. How does the electron-deficient nature of this compound influence its reactivity in Diels-Alder reactions?
Density functional theory (DFT) studies reveal that EMM’s electron-deficient diene system stabilizes polar transition states in reactions with electron-deficient ethylenes. Its dual ester groups lower the LUMO energy, enabling nucleophilic behavior toward strong electrophiles. This contrasts with traditional electron-rich dienes, allowing regioselective synthesis of cycloadducts .
Q. How can researchers resolve contradictions in catalytic efficiency between different Lewis acids for EMM-mediated reactions?
Conflicting catalytic outcomes (e.g., ZnBr₂ vs. Yb(OTf)₃ in cycloadditions) arise from variations in Lewis acid strength and steric effects. Methodological steps include:
- Electronic Analysis : Compare charge density via DFT to assess metal-ligand interactions.
- Steric Screening : Test bulky vs. compact catalysts (e.g., FeCl₃·Al₂O₃ vs. Sc(OTf)₃) .
- Analog Studies : Use dimethyl malonate as a structurally similar analog to infer reactivity patterns .
Q. What strategies optimize regioselective functionalization of this compound in heterocyclic synthesis?
- Directing Groups : Pyridine-containing derivatives (e.g., diethyl (6-methylpyridin-2-yl)aminomethylene-malonate) guide regioselectivity via coordination with transition metals .
- Solvent Effects : Polar aprotic solvents (e.g., 1,4-dioxane) enhance electrophilicity of the methylene group for nucleophilic attacks .
- Temperature Gradients : Higher temperatures (100–135°C) favor cyclization over side reactions in quinoline syntheses .
Q. How can researchers address solubility challenges in EMM-based derivatization for bioanalytical applications?
- Acidic Derivatization : Perform reactions under pH 2–3 to stabilize thiols (e.g., cysteine) and prevent oxidation .
- Co-solvent Systems : Use 1,2-dichloroethane/ethyl acetate mixtures to dissolve hydrophobic intermediates .
- Internal Standards : Isotope-labeled analogs (e.g., deuterated EMM) improve quantification accuracy in LC-MS/MS .
Q. Data Contradiction Analysis
Q. How should researchers interpret conflicting yields in EMM alkylation reactions under varying conditions?
Discrepancies in yields (e.g., 44.5% in dioxane vs. 50% in 1,2-dichloroethane) require systematic evaluation:
Properties
IUPAC Name |
diethyl 2-methylidenepropanedioate | |
---|---|---|
Source | PubChem | |
URL | https://pubchem.ncbi.nlm.nih.gov | |
Description | Data deposited in or computed by PubChem | |
InChI |
InChI=1S/C8H12O4/c1-4-11-7(9)6(3)8(10)12-5-2/h3-5H2,1-2H3 | |
Source | PubChem | |
URL | https://pubchem.ncbi.nlm.nih.gov | |
Description | Data deposited in or computed by PubChem | |
InChI Key |
BQHDXNZNSPVVKB-UHFFFAOYSA-N | |
Source | PubChem | |
URL | https://pubchem.ncbi.nlm.nih.gov | |
Description | Data deposited in or computed by PubChem | |
Canonical SMILES |
CCOC(=O)C(=C)C(=O)OCC | |
Source | PubChem | |
URL | https://pubchem.ncbi.nlm.nih.gov | |
Description | Data deposited in or computed by PubChem | |
Molecular Formula |
C8H12O4 | |
Source | PubChem | |
URL | https://pubchem.ncbi.nlm.nih.gov | |
Description | Data deposited in or computed by PubChem | |
Related CAS |
30329-60-3 | |
Record name | Propanedioic acid, 2-methylene-, 1,3-diethyl ester, homopolymer | |
Source | CAS Common Chemistry | |
URL | https://commonchemistry.cas.org/detail?cas_rn=30329-60-3 | |
Description | CAS Common Chemistry is an open community resource for accessing chemical information. Nearly 500,000 chemical substances from CAS REGISTRY cover areas of community interest, including common and frequently regulated chemicals, and those relevant to high school and undergraduate chemistry classes. This chemical information, curated by our expert scientists, is provided in alignment with our mission as a division of the American Chemical Society. | |
Explanation | The data from CAS Common Chemistry is provided under a CC-BY-NC 4.0 license, unless otherwise stated. | |
DSSTOX Substance ID |
DTXSID901033182 | |
Record name | Diethyl methylidenemalonate | |
Source | EPA DSSTox | |
URL | https://comptox.epa.gov/dashboard/DTXSID901033182 | |
Description | DSSTox provides a high quality public chemistry resource for supporting improved predictive toxicology. | |
Molecular Weight |
172.18 g/mol | |
Source | PubChem | |
URL | https://pubchem.ncbi.nlm.nih.gov | |
Description | Data deposited in or computed by PubChem | |
CAS No. |
3377-20-6 | |
Record name | 1,3-Diethyl 2-methylenepropanedioate | |
Source | CAS Common Chemistry | |
URL | https://commonchemistry.cas.org/detail?cas_rn=3377-20-6 | |
Description | CAS Common Chemistry is an open community resource for accessing chemical information. Nearly 500,000 chemical substances from CAS REGISTRY cover areas of community interest, including common and frequently regulated chemicals, and those relevant to high school and undergraduate chemistry classes. This chemical information, curated by our expert scientists, is provided in alignment with our mission as a division of the American Chemical Society. | |
Explanation | The data from CAS Common Chemistry is provided under a CC-BY-NC 4.0 license, unless otherwise stated. | |
Record name | Diethyl methylidenemalonate | |
Source | ChemIDplus | |
URL | https://pubchem.ncbi.nlm.nih.gov/substance/?source=chemidplus&sourceid=0003377206 | |
Description | ChemIDplus is a free, web search system that provides access to the structure and nomenclature authority files used for the identification of chemical substances cited in National Library of Medicine (NLM) databases, including the TOXNET system. | |
Record name | 3377-20-6 | |
Source | DTP/NCI | |
URL | https://dtp.cancer.gov/dtpstandard/servlet/dwindex?searchtype=NSC&outputformat=html&searchlist=18901 | |
Description | The NCI Development Therapeutics Program (DTP) provides services and resources to the academic and private-sector research communities worldwide to facilitate the discovery and development of new cancer therapeutic agents. | |
Explanation | Unless otherwise indicated, all text within NCI products is free of copyright and may be reused without our permission. Credit the National Cancer Institute as the source. | |
Record name | Propanedioic acid, 2-methylene-, 1,3-diethyl ester | |
Source | EPA Chemicals under the TSCA | |
URL | https://www.epa.gov/chemicals-under-tsca | |
Description | EPA Chemicals under the Toxic Substances Control Act (TSCA) collection contains information on chemicals and their regulations under TSCA, including non-confidential content from the TSCA Chemical Substance Inventory and Chemical Data Reporting. | |
Record name | Diethyl methylidenemalonate | |
Source | EPA DSSTox | |
URL | https://comptox.epa.gov/dashboard/DTXSID901033182 | |
Description | DSSTox provides a high quality public chemistry resource for supporting improved predictive toxicology. | |
Record name | Diethyl 2-methylenemalonate | |
Source | European Chemicals Agency (ECHA) | |
URL | https://echa.europa.eu/information-on-chemicals | |
Description | The European Chemicals Agency (ECHA) is an agency of the European Union which is the driving force among regulatory authorities in implementing the EU's groundbreaking chemicals legislation for the benefit of human health and the environment as well as for innovation and competitiveness. | |
Explanation | Use of the information, documents and data from the ECHA website is subject to the terms and conditions of this Legal Notice, and subject to other binding limitations provided for under applicable law, the information, documents and data made available on the ECHA website may be reproduced, distributed and/or used, totally or in part, for non-commercial purposes provided that ECHA is acknowledged as the source: "Source: European Chemicals Agency, http://echa.europa.eu/". Such acknowledgement must be included in each copy of the material. ECHA permits and encourages organisations and individuals to create links to the ECHA website under the following cumulative conditions: Links can only be made to webpages that provide a link to the Legal Notice page. | |
Retrosynthesis Analysis
AI-Powered Synthesis Planning: Our tool employs the Template_relevance Pistachio, Template_relevance Bkms_metabolic, Template_relevance Pistachio_ringbreaker, Template_relevance Reaxys, Template_relevance Reaxys_biocatalysis model, leveraging a vast database of chemical reactions to predict feasible synthetic routes.
One-Step Synthesis Focus: Specifically designed for one-step synthesis, it provides concise and direct routes for your target compounds, streamlining the synthesis process.
Accurate Predictions: Utilizing the extensive PISTACHIO, BKMS_METABOLIC, PISTACHIO_RINGBREAKER, REAXYS, REAXYS_BIOCATALYSIS database, our tool offers high-accuracy predictions, reflecting the latest in chemical research and data.
Strategy Settings
Precursor scoring | Relevance Heuristic |
---|---|
Min. plausibility | 0.01 |
Model | Template_relevance |
Template Set | Pistachio/Bkms_metabolic/Pistachio_ringbreaker/Reaxys/Reaxys_biocatalysis |
Top-N result to add to graph | 6 |
Feasible Synthetic Routes
Disclaimer and Information on In-Vitro Research Products
Please be aware that all articles and product information presented on BenchChem are intended solely for informational purposes. The products available for purchase on BenchChem are specifically designed for in-vitro studies, which are conducted outside of living organisms. In-vitro studies, derived from the Latin term "in glass," involve experiments performed in controlled laboratory settings using cells or tissues. It is important to note that these products are not categorized as medicines or drugs, and they have not received approval from the FDA for the prevention, treatment, or cure of any medical condition, ailment, or disease. We must emphasize that any form of bodily introduction of these products into humans or animals is strictly prohibited by law. It is essential to adhere to these guidelines to ensure compliance with legal and ethical standards in research and experimentation.