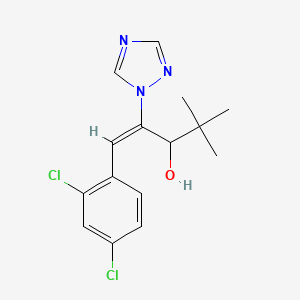
Diniconazole
Overview
Description
Diniconazole is a triazole fungicide known for its systemic action and ability to inhibit ergosterol biosynthesis in fungi. It is used to control a variety of fungal diseases, including powdery mildew, bunt, smut, and septoria leaf spot. The compound is characterized by its low aqueous solubility and low volatility, making it persistent in both soil and water systems .
Biochemical Analysis
Biochemical Properties
Diniconazole plays a crucial role in biochemical reactions by inhibiting the enzyme sterol 14α-demethylase (EC 1.14.13.70), which is involved in the biosynthesis of ergosterol, an essential component of fungal cell membranes . By inhibiting this enzyme, this compound disrupts the production of ergosterol, leading to the accumulation of toxic sterol intermediates and ultimately causing fungal cell death . The compound interacts with various biomolecules, including cytochrome P450 enzymes, which are responsible for its metabolism and detoxification .
Cellular Effects
This compound affects various types of cells and cellular processes. In fungal cells, it inhibits ergosterol biosynthesis, leading to altered cell membrane integrity and function . This disruption affects cell signaling pathways, gene expression, and cellular metabolism, ultimately resulting in fungal cell death . In plant cells, this compound has been shown to act as a growth retardant, affecting cell division and elongation . It also influences the expression of genes involved in stress responses and secondary metabolism .
Molecular Mechanism
At the molecular level, this compound exerts its effects by binding to the heme group of sterol 14α-demethylase, inhibiting its enzymatic activity . This binding prevents the conversion of lanosterol to ergosterol, leading to the accumulation of toxic sterol intermediates . Additionally, this compound can interact with other cytochrome P450 enzymes, affecting their activity and potentially leading to changes in the metabolism of other compounds . The inhibition of sterol 14α-demethylase also results in changes in gene expression, as the cell attempts to compensate for the disrupted ergosterol biosynthesis pathway .
Temporal Effects in Laboratory Settings
In laboratory settings, the effects of this compound change over time. The compound is relatively stable, with a biological half-life of approximately 6.2 days in plant tissues . Over time, this compound degrades into various metabolites, which may have different levels of activity and toxicity . Long-term exposure to this compound can lead to changes in cellular function, including alterations in gene expression and metabolic pathways . In in vitro studies, this compound has been shown to persist in both soil and water systems, indicating its potential for long-term environmental impact .
Dosage Effects in Animal Models
The effects of this compound vary with different dosages in animal models. At low doses, this compound is moderately toxic to mammals, with potential effects on reproduction and development . Higher doses can lead to more severe toxic effects, including liver and kidney damage . In birds and fish, this compound has been shown to be moderately toxic, with acute toxicity observed at higher concentrations . The compound’s toxicity is influenced by its ability to interact with cytochrome P450 enzymes, affecting the metabolism and detoxification of other compounds .
Metabolic Pathways
This compound is involved in several metabolic pathways, primarily through its interaction with cytochrome P450 enzymes . These enzymes are responsible for the oxidative metabolism of this compound, leading to the formation of various metabolites . The primary metabolic pathway involves the hydroxylation of the triazole ring and the subsequent formation of conjugates with glucuronic acid or sulfate . These metabolites are then excreted from the body through urine and feces . The inhibition of sterol 14α-demethylase by this compound also affects the ergosterol biosynthesis pathway, leading to the accumulation of toxic sterol intermediates .
Transport and Distribution
This compound is transported and distributed within cells and tissues through various mechanisms. It has low aqueous solubility and low volatility, which affects its distribution in the environment . In plant tissues, this compound is taken up through the roots and transported to other parts of the plant via the xylem . Within cells, this compound can interact with transporters and binding proteins, affecting its localization and accumulation . The compound’s systemic action allows it to provide both curative and protective effects against fungal pathogens .
Subcellular Localization
The subcellular localization of this compound is influenced by its chemical properties and interactions with cellular components. In fungal cells, this compound primarily localizes to the endoplasmic reticulum, where sterol 14α-demethylase is located . This localization allows this compound to effectively inhibit the enzyme and disrupt ergosterol biosynthesis . In plant cells, this compound can be found in various compartments, including the cytoplasm and vacuoles . The compound’s localization is influenced by targeting signals and post-translational modifications that direct it to specific cellular compartments .
Preparation Methods
Synthetic Routes and Reaction Conditions: Diniconazole is synthesized through a multi-step process involving the reaction of 2,4-dichlorobenzyl chloride with 1,2,4-triazole to form an intermediate, which is then reacted with tert-butylacetylene. The final step involves the addition of a hydroxyl group to the resulting compound .
Industrial Production Methods: Industrial production of this compound typically involves large-scale synthesis using similar reaction pathways as described above. The process is optimized for high yield and purity, often employing advanced techniques such as high-performance liquid chromatography (HPLC) for purification .
Chemical Reactions Analysis
Types of Reactions: Diniconazole undergoes various chemical reactions, including:
Oxidation: this compound can be oxidized to form this compound-M, a metabolite with similar fungicidal properties.
Reduction: The compound can be reduced under specific conditions, although this is less common in practical applications.
Substitution: this compound can undergo substitution reactions, particularly involving the triazole ring and the dichlorophenyl group.
Common Reagents and Conditions:
Oxidation: Common oxidizing agents include hydrogen peroxide and potassium permanganate.
Reduction: Reducing agents such as sodium borohydride can be used.
Substitution: Various nucleophiles can be employed for substitution reactions, depending on the desired product.
Major Products:
This compound-M: Formed through oxidation.
Substituted this compound Derivatives: Formed through substitution reactions.
Scientific Research Applications
Diniconazole has a wide range of applications in scientific research:
Chemistry: Used as a model compound for studying triazole fungicides and their interactions with fungal enzymes.
Medicine: Explored for its potential use in treating fungal infections in humans and animals.
Industry: Utilized in agriculture to protect crops from fungal diseases, thereby enhancing yield and quality.
Mechanism of Action
Diniconazole exerts its effects by inhibiting the enzyme sterol 14α-demethylase, which is involved in the biosynthesis of ergosterol, an essential component of fungal cell membranes. By blocking this enzyme, this compound disrupts the formation of ergosterol, leading to increased cellular permeability and ultimately the death of the fungal cell .
Comparison with Similar Compounds
Tebuconazole: Another triazole fungicide with a similar mechanism of action.
Metconazole: Shares structural similarities and is used for similar applications.
Fluconazole: A triazole antifungal used primarily in medicine
Uniqueness of Diniconazole: this compound is unique in its specific structural configuration, which allows for effective inhibition of sterol 14α-demethylase. Its persistence in soil and water systems also distinguishes it from some other triazole fungicides, making it particularly effective in certain agricultural settings .
Properties
CAS No. |
70217-36-6 |
---|---|
Molecular Formula |
C15H17Cl2N3O |
Molecular Weight |
326.2 g/mol |
IUPAC Name |
1-(2,4-dichlorophenyl)-4,4-dimethyl-2-(1,2,4-triazol-1-yl)pent-1-en-3-ol |
InChI |
InChI=1S/C15H17Cl2N3O/c1-15(2,3)14(21)13(20-9-18-8-19-20)6-10-4-5-11(16)7-12(10)17/h4-9,14,21H,1-3H3 |
InChI Key |
FBOUIAKEJMZPQG-UHFFFAOYSA-N |
Isomeric SMILES |
CC(C)(C)C(/C(=C/C1=C(C=C(C=C1)Cl)Cl)/N2C=NC=N2)O |
SMILES |
CC(C)(C)C(C(=CC1=C(C=C(C=C1)Cl)Cl)N2C=NC=N2)O |
Canonical SMILES |
CC(C)(C)C(C(=CC1=C(C=C(C=C1)Cl)Cl)N2C=NC=N2)O |
83657-24-3 | |
Pictograms |
Irritant; Environmental Hazard |
Synonyms |
1-(2,4-dichlorophenyl)-4,4-dimethyl-2-(1,2,4-triazol-1-yl)-1-penten-3-ol diniconazole S 3308 S-3308L |
Origin of Product |
United States |
Synthesis routes and methods I
Procedure details
Synthesis routes and methods II
Procedure details
Retrosynthesis Analysis
AI-Powered Synthesis Planning: Our tool employs the Template_relevance Pistachio, Template_relevance Bkms_metabolic, Template_relevance Pistachio_ringbreaker, Template_relevance Reaxys, Template_relevance Reaxys_biocatalysis model, leveraging a vast database of chemical reactions to predict feasible synthetic routes.
One-Step Synthesis Focus: Specifically designed for one-step synthesis, it provides concise and direct routes for your target compounds, streamlining the synthesis process.
Accurate Predictions: Utilizing the extensive PISTACHIO, BKMS_METABOLIC, PISTACHIO_RINGBREAKER, REAXYS, REAXYS_BIOCATALYSIS database, our tool offers high-accuracy predictions, reflecting the latest in chemical research and data.
Strategy Settings
Precursor scoring | Relevance Heuristic |
---|---|
Min. plausibility | 0.01 |
Model | Template_relevance |
Template Set | Pistachio/Bkms_metabolic/Pistachio_ringbreaker/Reaxys/Reaxys_biocatalysis |
Top-N result to add to graph | 6 |
Feasible Synthetic Routes
Disclaimer and Information on In-Vitro Research Products
Please be aware that all articles and product information presented on BenchChem are intended solely for informational purposes. The products available for purchase on BenchChem are specifically designed for in-vitro studies, which are conducted outside of living organisms. In-vitro studies, derived from the Latin term "in glass," involve experiments performed in controlled laboratory settings using cells or tissues. It is important to note that these products are not categorized as medicines or drugs, and they have not received approval from the FDA for the prevention, treatment, or cure of any medical condition, ailment, or disease. We must emphasize that any form of bodily introduction of these products into humans or animals is strictly prohibited by law. It is essential to adhere to these guidelines to ensure compliance with legal and ethical standards in research and experimentation.