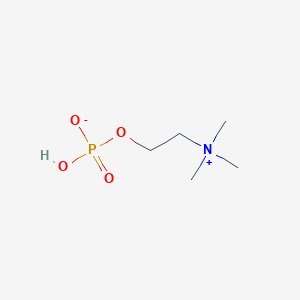
Phosphorylcholine
Overview
Description
Phosphorylcholine is a small molecule composed of a negatively charged phosphate group bonded to a positively charged choline group. It is the hydrophilic polar head group of some phospholipids, such as phosphatidylcholine and sphingomyelin . This compound is found in both prokaryotic and eukaryotic organisms and plays a crucial role in various biological processes, including cell membrane structure and function .
Preparation Methods
Synthetic Routes and Reaction Conditions: Phosphorylcholine can be synthesized through various methods. One common approach involves the free radical polymerization of ethylene monomers containing this compound groups and other ethylene monomers . Another method involves the copolymerization of 2-methacryloyloxyethyl this compound with alkyl methacrylates . These reactions typically require specific catalysts and controlled reaction conditions to ensure the desired product is obtained.
Industrial Production Methods: In industrial settings, this compound is often produced through large-scale polymerization processes. For example, this compound polymers can be coated on the surface of polymethylpentene hollow fiber membranes to improve their blood compatibility . These industrial methods involve precise control of reaction parameters to achieve high yields and consistent product quality.
Chemical Reactions Analysis
Types of Reactions: Phosphorylcholine undergoes various chemical reactions, including oxidation, reduction, and substitution. For instance, it can be oxidized to form this compound oxide or reduced to form this compound alcohol. Substitution reactions can occur when the choline group is replaced with other functional groups.
Common Reagents and Conditions: Common reagents used in the reactions of this compound include oxidizing agents like hydrogen peroxide, reducing agents like sodium borohydride, and various catalysts for substitution reactions. The reaction conditions, such as temperature and pH, are carefully controlled to optimize the reaction outcomes.
Major Products: The major products formed from the reactions of this compound depend on the specific reaction type. For example, oxidation reactions yield this compound oxide, while reduction reactions produce this compound alcohol. Substitution reactions result in various derivatives of this compound with different functional groups.
Scientific Research Applications
Phosphorylcholine has a wide range of scientific research applications across various fields:
Chemistry: In chemistry, this compound is used as a building block for the synthesis of complex molecules and polymers. Its unique structure allows for the creation of biocompatible materials with specific properties .
Biology: In biological research, this compound is studied for its role in cell membrane structure and function. It is also used as a marker for certain cellular processes and as a component of biomimetic membranes .
Medicine: In medicine, this compound is used in the development of drug delivery systems and medical devices. For example, this compound-coated stents are used to prevent coronary artery restenosis . Its biocompatibility and non-thrombogenic properties make it an ideal material for various medical applications.
Industry: In industrial applications, this compound polymers are used to improve the performance of membrane oxygenators, blood purification devices, and other biomedical devices . These polymers enhance the biocompatibility and functionality of the devices, making them more effective and reliable.
Mechanism of Action
Phosphorylcholine exerts its effects through various molecular mechanisms. It is a key component of cell membranes, where it contributes to membrane stability and fluidity . This compound also plays a role in cell signaling by interacting with specific receptors and enzymes. For example, it can activate protein kinase C, which is involved in various cellular processes . Additionally, this compound can modulate the immune response by interacting with immune cells and influencing their activity .
Comparison with Similar Compounds
Phosphorylcholine is similar to other choline-containing compounds, such as phosphatidylcholine and acetylcholine . it has unique properties that distinguish it from these compounds:
Phosphatidylcholine: Phosphatidylcholine is a major component of cell membranes and is involved in membrane-mediated cell signaling . Unlike this compound, phosphatidylcholine contains a glycerol backbone and two fatty acid chains.
Acetylcholine: Acetylcholine is a neurotransmitter that plays a crucial role in the nervous system . It is synthesized from choline and acetyl-CoA and is involved in transmitting nerve impulses. This compound, on the other hand, is not directly involved in neurotransmission.
Phosphoethanolamine: Phosphoethanolamine is another phospholipid head group that is structurally similar to this compound . it contains an ethanolamine group instead of a choline group, which gives it different chemical and biological properties.
Properties
IUPAC Name |
2-(trimethylazaniumyl)ethyl hydrogen phosphate | |
---|---|---|
Details | Computed by LexiChem 2.6.6 (PubChem release 2019.06.18) | |
Source | PubChem | |
URL | https://pubchem.ncbi.nlm.nih.gov | |
Description | Data deposited in or computed by PubChem | |
InChI |
InChI=1S/C5H14NO4P/c1-6(2,3)4-5-10-11(7,8)9/h4-5H2,1-3H3,(H-,7,8,9) | |
Details | Computed by InChI 1.0.5 (PubChem release 2019.06.18) | |
Source | PubChem | |
URL | https://pubchem.ncbi.nlm.nih.gov | |
Description | Data deposited in or computed by PubChem | |
InChI Key |
YHHSONZFOIEMCP-UHFFFAOYSA-N | |
Details | Computed by InChI 1.0.5 (PubChem release 2019.06.18) | |
Source | PubChem | |
URL | https://pubchem.ncbi.nlm.nih.gov | |
Description | Data deposited in or computed by PubChem | |
Canonical SMILES |
C[N+](C)(C)CCOP(=O)(O)[O-] | |
Details | Computed by OEChem 2.1.5 (PubChem release 2019.06.18) | |
Source | PubChem | |
URL | https://pubchem.ncbi.nlm.nih.gov | |
Description | Data deposited in or computed by PubChem | |
Molecular Formula |
C5H14NO4P | |
Details | Computed by PubChem 2.1 (PubChem release 2019.06.18) | |
Source | PubChem | |
URL | https://pubchem.ncbi.nlm.nih.gov | |
Description | Data deposited in or computed by PubChem | |
DSSTOX Substance ID |
DTXSID80983064 | |
Record name | 2-(Trimethylazaniumyl)ethyl hydrogen phosphate | |
Source | EPA DSSTox | |
URL | https://comptox.epa.gov/dashboard/DTXSID80983064 | |
Description | DSSTox provides a high quality public chemistry resource for supporting improved predictive toxicology. | |
Molecular Weight |
183.14 g/mol | |
Details | Computed by PubChem 2.1 (PubChem release 2021.05.07) | |
Source | PubChem | |
URL | https://pubchem.ncbi.nlm.nih.gov | |
Description | Data deposited in or computed by PubChem | |
CAS No. |
645-84-1 | |
Record name | Cholinephosphoric acid | |
Source | CAS Common Chemistry | |
URL | https://commonchemistry.cas.org/detail?cas_rn=645-84-1 | |
Description | CAS Common Chemistry is an open community resource for accessing chemical information. Nearly 500,000 chemical substances from CAS REGISTRY cover areas of community interest, including common and frequently regulated chemicals, and those relevant to high school and undergraduate chemistry classes. This chemical information, curated by our expert scientists, is provided in alignment with our mission as a division of the American Chemical Society. | |
Explanation | The data from CAS Common Chemistry is provided under a CC-BY-NC 4.0 license, unless otherwise stated. | |
Record name | Phosphorylcholine | |
Source | ChemIDplus | |
URL | https://pubchem.ncbi.nlm.nih.gov/substance/?source=chemidplus&sourceid=0000645841 | |
Description | ChemIDplus is a free, web search system that provides access to the structure and nomenclature authority files used for the identification of chemical substances cited in National Library of Medicine (NLM) databases, including the TOXNET system. | |
Record name | 2-(Trimethylazaniumyl)ethyl hydrogen phosphate | |
Source | EPA DSSTox | |
URL | https://comptox.epa.gov/dashboard/DTXSID80983064 | |
Description | DSSTox provides a high quality public chemistry resource for supporting improved predictive toxicology. | |
Record name | PHOSPHORYLCHOLINE | |
Source | FDA Global Substance Registration System (GSRS) | |
URL | https://gsrs.ncats.nih.gov/ginas/app/beta/substances/BRP7TI555O | |
Description | The FDA Global Substance Registration System (GSRS) enables the efficient and accurate exchange of information on what substances are in regulated products. Instead of relying on names, which vary across regulatory domains, countries, and regions, the GSRS knowledge base makes it possible for substances to be defined by standardized, scientific descriptions. | |
Explanation | Unless otherwise noted, the contents of the FDA website (www.fda.gov), both text and graphics, are not copyrighted. They are in the public domain and may be republished, reprinted and otherwise used freely by anyone without the need to obtain permission from FDA. Credit to the U.S. Food and Drug Administration as the source is appreciated but not required. | |
Retrosynthesis Analysis
AI-Powered Synthesis Planning: Our tool employs the Template_relevance Pistachio, Template_relevance Bkms_metabolic, Template_relevance Pistachio_ringbreaker, Template_relevance Reaxys, Template_relevance Reaxys_biocatalysis model, leveraging a vast database of chemical reactions to predict feasible synthetic routes.
One-Step Synthesis Focus: Specifically designed for one-step synthesis, it provides concise and direct routes for your target compounds, streamlining the synthesis process.
Accurate Predictions: Utilizing the extensive PISTACHIO, BKMS_METABOLIC, PISTACHIO_RINGBREAKER, REAXYS, REAXYS_BIOCATALYSIS database, our tool offers high-accuracy predictions, reflecting the latest in chemical research and data.
Strategy Settings
Precursor scoring | Relevance Heuristic |
---|---|
Min. plausibility | 0.01 |
Model | Template_relevance |
Template Set | Pistachio/Bkms_metabolic/Pistachio_ringbreaker/Reaxys/Reaxys_biocatalysis |
Top-N result to add to graph | 6 |
Feasible Synthetic Routes
Disclaimer and Information on In-Vitro Research Products
Please be aware that all articles and product information presented on BenchChem are intended solely for informational purposes. The products available for purchase on BenchChem are specifically designed for in-vitro studies, which are conducted outside of living organisms. In-vitro studies, derived from the Latin term "in glass," involve experiments performed in controlled laboratory settings using cells or tissues. It is important to note that these products are not categorized as medicines or drugs, and they have not received approval from the FDA for the prevention, treatment, or cure of any medical condition, ailment, or disease. We must emphasize that any form of bodily introduction of these products into humans or animals is strictly prohibited by law. It is essential to adhere to these guidelines to ensure compliance with legal and ethical standards in research and experimentation.