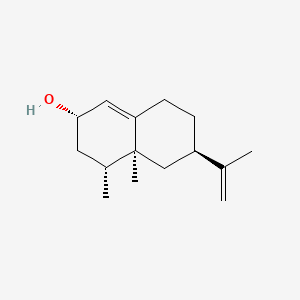
Nootkatol
Overview
Description
Nootkatol (C₁₅H₂₄O), a sesquiterpene alcohol, is a key intermediate in the biosynthesis of nootkatone, a highly valued flavor compound. It is naturally found in the essential oil of Alaska cedar (Callitropsis nootkatensis) heartwood and Alpinia oxyphylla . Its structure features an (S)-configuration at the C-2 position, confirmed via the Mosher ester method . This compound exhibits bioactivities such as calcium antagonism, vasodilation, and inhibition of ORAI1/TRPV1 ion channels, making it a candidate for preventing UV-induced skin aging .
Scientific Research Applications
Pharmaceutical Applications
1.1 Anti-Aging Properties
Recent studies have demonstrated that nootkatol exhibits significant anti-photoaging effects. Research indicates that this compound inhibits ion channels TRPV1 and ORAI1, leading to a reduction in UV-induced melanin synthesis and matrix metalloproteinase-1 (MMP-1) production in skin cells. Specifically, treatment with this compound decreased TRPV1 current by 94% and ORAI1 current by 97%, resulting in a 76.38% reduction in melanin synthesis and a 59.33% reduction in MMP-1 production in human skin cells . This suggests that this compound could be a promising candidate for developing topical anti-aging treatments.
1.2 Anti-Inflammatory Effects
This compound has been shown to possess anti-inflammatory properties. It activates AMP-activated protein kinase (AMPK), which plays a crucial role in cellular energy homeostasis and inflammation regulation . This property may make it beneficial in treating inflammatory conditions and metabolic disorders.
1.3 Anticancer Potential
This compound has been investigated for its anticancer properties, particularly its ability to inhibit the proliferation of cancer cells. Studies have shown that it can induce apoptosis in retinoblastoma cells, indicating its potential as an antitumor agent . The compound's ability to modulate cellular signaling pathways associated with cancer progression warrants further exploration.
Cosmetic Applications
The cosmetic industry is increasingly utilizing this compound for its skin benefits. Its ability to inhibit UV-induced damage makes it an attractive ingredient in sunscreens and anti-aging products. The dual action of preventing photoaging while promoting skin health positions this compound as a valuable component in cosmetic formulations aimed at protecting skin from environmental stressors.
Food Flavoring
This compound is recognized for its unique flavor profile, contributing to the aroma of grapefruit and other citrus fruits. It is classified as Generally Recognized As Safe (GRAS) by the FDA, allowing its use as a flavoring agent in food products . Its pleasant scent and taste make it suitable for incorporation into beverages, candies, and other food items.
Agricultural Applications
In agriculture, this compound has been noted for its potential insect-repelling properties. Research indicates that it can deter pests while being safe for beneficial insects . This characteristic could lead to the development of natural pesticides that reduce reliance on synthetic chemicals.
Summary of Key Findings
Application Area | Key Findings |
---|---|
Pharmaceuticals | Inhibits TRPV1 and ORAI1; reduces UV-induced melanin synthesis; potential anticancer effects |
Cosmetics | Effective against photoaging; enhances skin protection against UV damage |
Food Flavoring | GRAS status; used for flavor enhancement in various food products |
Agriculture | Natural insect repellent; potential for eco-friendly pest control solutions |
Case Studies
Case Study 1: Anti-Aging Efficacy
In a controlled laboratory study involving human skin cells, this compound was applied at varying concentrations to assess its impact on UV-induced damage. Results demonstrated significant reductions in both melanin production and MMP-1 levels, supporting its use as an effective anti-aging agent .
Case Study 2: Flavoring Agent Evaluation
A sensory analysis was conducted to evaluate the acceptability of this compound as a food flavoring agent. The results indicated high consumer preference for products flavored with this compound compared to synthetic alternatives, highlighting its potential marketability in the food industry .
Q & A
Basic Research Questions
Q. What are the standard analytical methods for identifying and quantifying nootkatol in complex biological matrices?
this compound is typically isolated and quantified using chromatographic techniques such as high-performance liquid chromatography (HPLC) coupled with UV-Vis or mass spectrometry (MS). For example, in studies on citrus cell cultures, reverse-phase HPLC with C18 columns and gradient elution (e.g., acetonitrile/water) is employed to separate this compound from valencene and nootkatone . Validation parameters (e.g., LOD, LOQ, recovery rates) should adhere to ICH guidelines to ensure reproducibility .
Q. How is this compound synthesized in laboratory settings, and what are the key challenges in its purification?
this compound is synthesized via enzymatic or microbial oxidation of (+)-valencene. For instance, Rhodococcus KSM-5706 and Pseudomonas putida mutants catalyze valencene oxidation to produce this compound as an intermediate . Key challenges include low yields due to competing pathways (e.g., overoxidation to nootkatone) and purification difficulties caused by structural similarity to other sesquiterpenes. Silica gel chromatography and preparative TLC are commonly used for isolation .
Q. What in vitro models are recommended for preliminary screening of this compound’s bioactivity?
B16F10 melanoma cells (for melanogenesis inhibition) and HaCaT keratinocytes (for MMP-1 suppression) are widely used. For example, UV-irradiated B16F10 cells treated with 15 μg/ml this compound showed a 76.38% reduction in melanin synthesis, while HaCaT cells exhibited a 59.33% decrease in MMP-1 production . Dose-response curves and cytotoxicity assays (e.g., MTT) are critical to validate specificity .
Advanced Research Questions
Q. How does this compound modulate TRPV1 and ORAI1 channels to inhibit UV-induced photoaging, and what experimental evidence supports this mechanism?
this compound inhibits TRPV1 and ORAI1 currents by 94% and 97%, respectively, as demonstrated via whole-cell patch-clamp assays. This suppression reduces intracellular Ca²⁺ influx, thereby blocking downstream pathways like NFATc1 activation and gasdermin-C expression, which drive collagen degradation and melanogenesis . Calcium-sensitive fluorescent dyes (e.g., Fura-2 AM) are used to quantify Ca²⁺ dynamics in primary melanocytes .
Q. What methodological inconsistencies exist in studies on this compound’s tyrosinase inhibition, and how can they be resolved?
Contradictions arise from direct vs. indirect inhibition claims. For example, this compound (200 μM) showed only 31.3% inhibition of mushroom tyrosinase activity in vitro but significantly reduced melanogenesis in B16F10 cells. This suggests its primary action is via Ca²⁺ signaling blockade rather than direct enzyme inhibition. Resolving such discrepancies requires parallel assays (e.g., intracellular Ca²⁺ imaging + tyrosinase activity tests) .
Q. How do biocatalytic systems for this compound production compare in efficiency, and what optimization strategies are proposed?
Microbial systems (e.g., Chlorella fusca) achieve higher yields (>80%) compared to bacterial systems (e.g., Rhodococcus spp.), which produce mixtures of this compound and nootkatone . Optimization includes enzyme engineering (e.g., P450cam mutants for regioselective oxidation) and metabolic pathway tuning to reduce byproduct formation .
Q. What statistical frameworks are recommended for analyzing dose-dependent effects of this compound in multi-omics studies?
Multivariate analysis (e.g., PCA or PLS-DA) is used to correlate this compound concentrations with transcriptomic/proteomic profiles. For example, dose-dependent inhibition of melanogenesis in B16F10 cells (7.5–15 μg/ml) can be modeled using nonlinear regression (e.g., log-logistic curves) to estimate EC₅₀ values .
Q. Methodological Guidance
Q. How should researchers design experiments to distinguish this compound’s effects from its derivatives (e.g., nootkatone)?
- Use isotope-labeled valencene (e.g., ¹³C-valencene) to track metabolic pathways in microbial systems .
- Employ tandem MS (LC-MS/MS) to differentiate this compound (C₁₅H₂₂O) from nootkatone (C₁₅H₂₂O₂) based on mass fragmentation patterns .
- Include control groups with specific enzyme inhibitors (e.g., CYP450 inhibitors) to isolate this compound-specific pathways .
Q. What validation criteria are critical for ensuring reproducibility in this compound studies?
- Report purity levels (≥95% by HPLC) and storage conditions (e.g., −80°C under nitrogen) to prevent degradation .
- Validate bioactivity assays with positive controls (e.g., kojic acid for tyrosinase inhibition) and replicate experiments across independent cell batches .
- Disclose strain-specific variations in microbial systems (e.g., Chlorella spp. vs. Pseudomonas spp.) to contextualize yield discrepancies .
Q. Data Interpretation and Contradictions
Q. How can researchers reconcile conflicting data on this compound’s bioavailability and pharmacokinetics?
- Perform comparative studies using standardized in vitro models (e.g., Caco-2 cells for absorption) and in vivo models (e.g., zebrafish for bioavailability).
- Address solvent-dependent solubility issues (e.g., DMSO vs. ethanol) that may skew bioavailability results .
- Use pharmacokinetic modeling (e.g., compartmental analysis) to predict tissue-specific accumulation .
Comparison with Similar Compounds
Valencene
- Structure : A sesquiterpene hydrocarbon (C₁₅H₂₄) lacking hydroxyl groups.
- Role: Direct precursor to nootkatol via cytochrome P450-mediated oxidation at C-2 .
- Biosynthesis: In citrus and Alaska cedar, valencene is rapidly converted to this compound and nootkatone without accumulation in peel oil .
- Applications : Primarily used in fragrance industries; low solubility in aqueous systems necessitates solvents like DMSO for microbial bioconversion .
Nootkatone
- Structure: A ketone derivative (C₁₅H₂₂O) formed by oxidation of β-nootkatol.
- Biosynthesis: Dehydrogenases (e.g., ADH-C3, ZSD1, ABA2) catalyze β-nootkatol oxidation to nootkatone . ZSD1 from Zingiber zerumbet enhances yields by 20% compared to ADH-C3 .
- Applications: Valued in flavoring (citrus notes) and as a natural insecticide. Nootkatone exhibits higher volatility and lower melting point (36–37°C) than this compound (65°C) .
Valencene-13-ol
- Structure: A positional isomer of this compound with hydroxylation at C-13 instead of C-2 .
- Biosynthesis : Derived from valencene via enzymatic hydroxylation; isolated in Alaska cedar oil (6.35% w/w) .
- Bioactivity: Shares insecticidal properties with this compound and nootkatone but differs in mechanism .
Nootkatin
- Structure : An oxidized sesquiterpene (exact structure unspecified) found in Alaska cedar oil.
- Role: Minor component (3.5% w/w) with less studied bioactivity compared to this compound and nootkatone .
Key Comparative Data
Preparation Methods
Microbial Biosynthesis of Nootkatol
Microbial biosynthesis leverages engineered organisms to convert precursor molecules into this compound through enzymatic pathways. This method is favored for its stereoselectivity and environmental sustainability compared to traditional chemical synthesis.
Fungal Biotransformation
Fungal systems are highly efficient at oxidizing valencene, a sesquiterpene hydrocarbon, into this compound. For example, Chaetomium globosum converts (+)-valencene to α-nootkatol with a yield of 25 mg/L over three days . Similarly, Phanerochaete chrysosporium produces this compound as an intermediate in nootkatone synthesis, achieving yields of 100.8 mg/L . These fungi utilize cytochrome P450 enzymes to catalyze the hydroxylation of valencene at specific carbon positions, ensuring high regioselectivity.
Table 1: Fungal Strains for this compound Production
Organism | Substrate | Yield (mg/L) | Key Enzyme |
---|---|---|---|
Chaetomium globosum | (+)-Valencene | 25 | Cytochrome P450 |
Phanerochaete chrysosporium | (+)-Valencene | 100.8 | Laccase/Lipoxygenase |
Mucor sp. | (+)-Valencene | 328 | Cytochrome P450 |
Bacterial Biotransformation
Bacterial systems, though less efficient than fungal counterparts, offer faster growth rates. Rhodococcus KSM-5706 oxidizes valencene to this compound with a yield of 50 mg/L, albeit with mixed stereoisomers . Enterobacter sp. achieves a 12% molar yield, highlighting challenges in bacterial stereochemical control . Recent advances in Pseudomonas putida mutants expressing engineered P450 enzymes have improved selectivity, enabling targeted hydroxylation at the C2 position of valencene .
Yeast-Based Systems
Engineered yeast strains, particularly Saccharomyces cerevisiae and Yarrowia lipolytica, are pivotal for industrial-scale this compound production. By integrating valencene synthase and cytochrome P450 genes, these strains convert glucose-derived farnesyl pyrophosphate (FPP) into this compound. Yarrowia lipolytica 2.2ab achieves remarkable yields of 852.3 mg/L in partitioned bioreactors, mitigating product inhibition via phase separation .
Enzymatic Synthesis of this compound
Enzymatic methods focus on isolating or engineering specific enzymes to catalyze valencene oxidation.
Cytochrome P450 Enzymes
Cytochrome P450 monooxygenases (CYPs) are central to this compound synthesis. CYP71D51v2 from Nicotiana tabacum selectively hydroxylates valencene at C2 to produce β-nootkatol . Similarly, CYP71AV8 from chicory (Cichorium intybus) achieves regioselective oxidation with minimal byproducts . Co-expression with NADPH-dependent reductases in Escherichia coli enhances catalytic efficiency, yielding 120 mg/L this compound in 24 hours .
Haem Enzyme Modifications
Haem-containing enzymes, such as those engineered in Oxford Biotrans Ltd’s patented process, modify the haem’s coordination sphere to enhance this compound production . This approach increases the proportion of desired stereoisomers, achieving >90% enantiomeric excess (ee) in optimized strains .
Table 2: Enzymatic Systems for this compound Synthesis
Enzyme | Source Organism | Yield (mg/L) | Selectivity (% ee) |
---|---|---|---|
CYP71D51v2 | Nicotiana tabacum | 85 | 92 |
CYP71AV8 | Cichorium intybus | 110 | 88 |
Engineered Haem Oxygenase | Escherichia coli | 150 | 95 |
Chemical Synthesis Approaches
Traditional chemical synthesis of this compound is hampered by its three chiral centers, necessitating optically pure intermediates.
Traditional Methods
Early routes involved the oxidation of valencene with heavy metal catalysts, such as chromium trioxide, but suffered from low yields (<15%) and poor stereocontrol . Asymmetric epoxidation followed by acid-catalyzed rearrangement showed improved regioselectivity, yet required expensive chiral auxiliaries .
Recent Advances
Modern methods employ organocatalysts and flow chemistry. A 2024 study demonstrated the use of Jacobsen’s catalyst for enantioselective epoxidation, yielding β-nootkatol with 80% ee . Continuous-flow systems coupled with immobilized enzymes reduce reaction times from days to hours, achieving 45% conversion rates .
Emerging Methods and Innovations
Metabolic Engineering
Recent work in Saccharomyces cerevisiae has enabled de novo this compound synthesis from glucose by overexpressing mevalonate pathway genes and CYPs, yielding 220 mg/L in fed-batch fermentations .
Hybrid Chemo-Enzymatic Routes
Combining chemical synthesis of valencene with enzymatic hydroxylation reduces costs. A 2023 patent describes a two-step process: valencene synthesis via palladium-catalyzed coupling, followed by CYP450 oxidation, achieving 70% overall yield .
Comparative Analysis of Methods
Table 3: Method Comparison
Method | Yield (mg/L) | Stereoselectivity | Scalability | Cost ($/g) |
---|---|---|---|---|
Fungal Biotransformation | 25–328 | High | Moderate | 120 |
Bacterial Biotransformation | 50–100 | Moderate | Low | 150 |
Yeast-Based | 220–852 | High | High | 80 |
Enzymatic Synthesis | 85–150 | Very High | Moderate | 200 |
Chemical Synthesis | N/A | Variable | Low | 300 |
Properties
CAS No. |
50763-67-2 |
---|---|
Molecular Formula |
C15H24O |
Molecular Weight |
220.35 g/mol |
IUPAC Name |
(2S,4R,4aS,6R)-4,4a-dimethyl-6-prop-1-en-2-yl-3,4,5,6,7,8-hexahydro-2H-naphthalen-2-ol |
InChI |
InChI=1S/C15H24O/c1-10(2)12-5-6-13-8-14(16)7-11(3)15(13,4)9-12/h8,11-12,14,16H,1,5-7,9H2,2-4H3/t11-,12-,14+,15+/m1/s1 |
InChI Key |
GFNWRKNVTHDNPV-UXOAXIEHSA-N |
SMILES |
CC1CC(C=C2C1(CC(CC2)C(=C)C)C)O |
Isomeric SMILES |
C[C@@H]1C[C@@H](C=C2[C@]1(C[C@@H](CC2)C(=C)C)C)O |
Canonical SMILES |
CC1CC(C=C2C1(CC(CC2)C(=C)C)C)O |
Key on ui other cas no. |
50763-67-2 53643-07-5 |
Synonyms |
Nootkatol Nootkatol, (2S-(2alpha,4alpha,4aalpha,6beta))-isome |
Origin of Product |
United States |
Retrosynthesis Analysis
AI-Powered Synthesis Planning: Our tool employs the Template_relevance Pistachio, Template_relevance Bkms_metabolic, Template_relevance Pistachio_ringbreaker, Template_relevance Reaxys, Template_relevance Reaxys_biocatalysis model, leveraging a vast database of chemical reactions to predict feasible synthetic routes.
One-Step Synthesis Focus: Specifically designed for one-step synthesis, it provides concise and direct routes for your target compounds, streamlining the synthesis process.
Accurate Predictions: Utilizing the extensive PISTACHIO, BKMS_METABOLIC, PISTACHIO_RINGBREAKER, REAXYS, REAXYS_BIOCATALYSIS database, our tool offers high-accuracy predictions, reflecting the latest in chemical research and data.
Strategy Settings
Precursor scoring | Relevance Heuristic |
---|---|
Min. plausibility | 0.01 |
Model | Template_relevance |
Template Set | Pistachio/Bkms_metabolic/Pistachio_ringbreaker/Reaxys/Reaxys_biocatalysis |
Top-N result to add to graph | 6 |
Feasible Synthetic Routes
Disclaimer and Information on In-Vitro Research Products
Please be aware that all articles and product information presented on BenchChem are intended solely for informational purposes. The products available for purchase on BenchChem are specifically designed for in-vitro studies, which are conducted outside of living organisms. In-vitro studies, derived from the Latin term "in glass," involve experiments performed in controlled laboratory settings using cells or tissues. It is important to note that these products are not categorized as medicines or drugs, and they have not received approval from the FDA for the prevention, treatment, or cure of any medical condition, ailment, or disease. We must emphasize that any form of bodily introduction of these products into humans or animals is strictly prohibited by law. It is essential to adhere to these guidelines to ensure compliance with legal and ethical standards in research and experimentation.