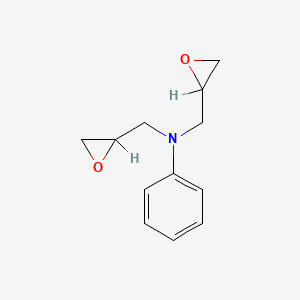
Diglycidylaniline
Overview
Description
Diglycidylaniline, also known as N,N-diglycidylaniline, is an organic compound belonging to the glycidyl family. It is characterized by the presence of two epoxy groups attached to an aniline ring. The compound has the molecular formula C₁₂H₁₅NO₂ and a molecular weight of 205.25 g/mol . This compound is primarily used as a reactive diluent in epoxy resin systems to reduce viscosity and enhance the mechanical properties of the resins .
Mechanism of Action
Target of Action
Diglycidylaniline, also known as N,N-bis(oxiran-2-ylmethyl)aniline , is an aromatic organic chemical in the glycidyl compound family . It is primarily used to reduce the viscosity of epoxy resin systems . .
Mode of Action
It is known that many glycidyl ethers, including this compound, are manufactured by the addition of epichlorohydrin to a species with the aid of a lewis acid as a catalyst to form a halohydrin . This process is followed by washing with sodium hydroxide in a dehydrochlorination reaction .
Biochemical Pathways
It is known that the use of the diluent in epoxy systems affects the mechanical properties and microstructure of epoxy resins
Pharmacokinetics
It is known that this compound has a molecular weight of 20525 and a density of 1.153 g/mL at 25 °C .
Result of Action
It is known that the kinetics of cure of this compound with epoxy resin networks have been studied
Action Environment
It is known that many factors can affect the efficacy of a compound, including temperature, ph, and the presence of other chemicals
Biochemical Analysis
Biochemical Properties
Diglycidylaniline plays a significant role in biochemical reactions due to its reactive epoxide groups. These groups can interact with various enzymes, proteins, and other biomolecules. One of the key interactions is with enzymes involved in the curing of epoxy resin networks, where this compound acts as a cross-linking agent . This interaction enhances the mechanical properties and microstructure of the resulting epoxy resins . Additionally, this compound can interact with proteins and other biomolecules through its epoxide groups, forming covalent bonds that can alter the structure and function of these biomolecules .
Cellular Effects
The effects of this compound on various types of cells and cellular processes are profound. It can influence cell function by interacting with cellular proteins and enzymes, leading to changes in cell signaling pathways, gene expression, and cellular metabolism. For instance, this compound has been shown to affect the kinetics of cure in epoxy resin networks, which can impact cellular processes such as cell adhesion and proliferation . Moreover, the reactive nature of its epoxide groups can lead to the formation of covalent bonds with cellular proteins, potentially altering their function and affecting cellular homeostasis .
Molecular Mechanism
At the molecular level, this compound exerts its effects through its reactive epoxide groups. These groups can form covalent bonds with nucleophilic sites on biomolecules, such as amino acids in proteins or nucleotides in DNA . This binding can result in enzyme inhibition or activation, depending on the specific biomolecule involved. For example, this compound can inhibit enzymes involved in DNA repair, leading to changes in gene expression and cellular responses to DNA damage . Additionally, the formation of covalent bonds with proteins can alter their structure and function, impacting various cellular processes .
Temporal Effects in Laboratory Settings
In laboratory settings, the effects of this compound can change over time due to its stability and degradation. Studies have shown that this compound is relatively stable under standard laboratory conditions, but it can degrade over time, especially when exposed to heat or light . This degradation can lead to changes in its reactivity and its effects on cellular function. Long-term exposure to this compound in in vitro or in vivo studies has been associated with alterations in cellular processes, such as changes in cell proliferation and gene expression .
Dosage Effects in Animal Models
The effects of this compound vary with different dosages in animal models. At low doses, this compound can act as a reactive diluent, enhancing the properties of epoxy resins without causing significant toxic effects . At higher doses, this compound can exhibit toxic effects, including cellular damage and alterations in metabolic processes . Studies in animal models have shown that high doses of this compound can lead to adverse effects such as liver and kidney damage, highlighting the importance of dosage control in its applications .
Metabolic Pathways
This compound is involved in various metabolic pathways, primarily through its interactions with enzymes and cofactors. The epoxide groups in this compound can be metabolized by enzymes such as epoxide hydrolases, leading to the formation of diols . These metabolic reactions can affect the overall metabolic flux and levels of metabolites in cells. Additionally, this compound can influence the activity of enzymes involved in other metabolic pathways, potentially altering cellular metabolism and energy production .
Transport and Distribution
Within cells and tissues, this compound is transported and distributed through interactions with transporters and binding proteins. The compound can be taken up by cells through passive diffusion or active transport mechanisms . Once inside the cells, this compound can bind to various proteins, affecting its localization and accumulation. These interactions can influence the compound’s activity and its effects on cellular processes .
Subcellular Localization
The subcellular localization of this compound is influenced by its chemical properties and interactions with cellular components. The compound can be directed to specific cellular compartments, such as the endoplasmic reticulum or mitochondria, through targeting signals or post-translational modifications . These localizations can affect the activity and function of this compound, as well as its interactions with other biomolecules within these compartments .
Preparation Methods
Diglycidylaniline is typically synthesized through the reaction of aniline with epichlorohydrin in the presence of a Lewis acid catalyst. The reaction proceeds via the formation of a halohydrin intermediate, which is subsequently dehydrochlorinated using sodium hydroxide to yield the final product . The industrial production of this compound follows a similar route, ensuring high purity and yield through optimized reaction conditions and purification steps .
Chemical Reactions Analysis
Diglycidylaniline undergoes various chemical reactions, including:
Epoxy Ring Opening: The epoxy groups in this compound can undergo ring-opening reactions with nucleophiles such as amines, alcohols, and thiols.
Cyclization: The reaction between this compound and aromatic amines can lead to the formation of cyclic products.
Polymerization: this compound can be used as a monomer in the synthesis of epoxy resins.
Scientific Research Applications
Diglycidylaniline has a wide range of applications in scientific research, including:
Polymer Chemistry: It is used as a reactive diluent in epoxy resin formulations to improve the processing and mechanical properties of the resins.
Material Science: this compound is utilized in the development of advanced materials such as composites, adhesives, and coatings.
Biomedical Research:
Comparison with Similar Compounds
Diglycidylaniline can be compared with other glycidyl compounds, such as:
Bisphenol A Diglycidyl Ether (BADGE): Both compounds contain epoxy groups, but BADGE has a bisphenol A backbone, which imparts different mechanical and thermal properties to the resulting polymers.
Epichlorohydrin: While epichlorohydrin is a precursor in the synthesis of this compound, it lacks the aromatic ring and has different reactivity and applications.
N,N-Diglycidyl-4-glycidyloxyaniline: This compound has an additional glycidyloxy group, which can lead to higher crosslink density and different mechanical properties in the resulting polymers.
This compound stands out due to its unique combination of epoxy groups and aniline ring, which provides a balance of reactivity and mechanical properties suitable for various applications.
Biological Activity
Diglycidylaniline (DGA) is a compound that has garnered significant attention due to its unique chemical properties and potential applications in various fields, including materials science and biomedical engineering. This article delves into the biological activity of DGA, focusing on its biochemical interactions, effects on cellular processes, and implications for medical applications.
Chemical Structure and Properties
This compound is characterized by its epoxy groups that can form covalent bonds with nucleophilic sites on proteins and enzymes. This bifunctional nature allows DGA to participate in various biochemical reactions, making it a versatile compound in both industrial and biological contexts.
Covalent Bond Formation
DGA's epoxy groups enable it to react with amino acids in proteins, leading to crosslinking. This crosslinking can stabilize protein structures, enhancing their thermal and chemical stability. The amine groups present in DGA also participate in hydrogen bonding and ionic interactions, further contributing to the stability and functionality of biomolecules .
Enzyme Activity Modulation
Research indicates that DGA can influence enzyme activity through covalent modification. Depending on the specific enzyme and the site of modification, DGA can either inhibit or activate enzymatic functions. This modulation can have downstream effects on metabolic pathways, influencing cellular metabolism and overall function .
Impact on Cell Signaling
DGA has been shown to affect various cellular processes, including cell signaling pathways. By interacting with key signaling proteins, DGA can lead to changes in gene expression and metabolic activity. For instance, the crosslinking of proteins by DGA alters the activity of enzymes involved in critical metabolic pathways .
Dosage-Dependent Effects
The biological effects of DGA vary significantly with dosage. At low concentrations, it enhances protein stability without causing toxicity. However, at higher concentrations, it may induce toxic effects such as cell death and tissue damage due to excessive crosslinking of proteins .
Dosage Level | Effect | Outcome |
---|---|---|
Low | Enhanced protein stability | Increased functionality |
Moderate | Normal cellular function | No significant adverse effects |
High | Toxicity | Cell death and tissue damage |
Case Studies
- Cellular Metabolism : A study demonstrated that DGA influences the metabolic pathways by inhibiting enzymes responsible for protein degradation. This leads to an accumulation of protein aggregates within cells, potentially disrupting normal cellular functions .
- Animal Models : In animal studies, varying doses of DGA were administered to assess its impact on tissue integrity and cellular health. Results indicated that while low doses promoted cellular stability, high doses resulted in significant tissue damage due to extensive protein crosslinking .
- Long-Term Effects : Longitudinal studies have shown that even after degradation of DGA, initial interactions with biomolecules can have lasting consequences on gene expression and metabolic activity within cells .
Applications in Biomedical Engineering
DGA's ability to form strong covalent bonds makes it a valuable component in the synthesis of biocompatible materials used for medical devices and drug delivery systems. Its role as a crosslinking agent is crucial in developing hydrogels and tissue engineering scaffolds that require stable yet flexible structures .
Application | Description |
---|---|
Medical Devices | Used as a crosslinker for biocompatible materials |
Drug Delivery Systems | Forms stable networks for controlled release |
Tissue Engineering Scaffolds | Enhances structural integrity while maintaining flexibility |
Properties
IUPAC Name |
N,N-bis(oxiran-2-ylmethyl)aniline | |
---|---|---|
Source | PubChem | |
URL | https://pubchem.ncbi.nlm.nih.gov | |
Description | Data deposited in or computed by PubChem | |
InChI |
InChI=1S/C12H15NO2/c1-2-4-10(5-3-1)13(6-11-8-14-11)7-12-9-15-12/h1-5,11-12H,6-9H2 | |
Source | PubChem | |
URL | https://pubchem.ncbi.nlm.nih.gov | |
Description | Data deposited in or computed by PubChem | |
InChI Key |
JAYXSROKFZAHRQ-UHFFFAOYSA-N | |
Source | PubChem | |
URL | https://pubchem.ncbi.nlm.nih.gov | |
Description | Data deposited in or computed by PubChem | |
Canonical SMILES |
C1C(O1)CN(CC2CO2)C3=CC=CC=C3 | |
Source | PubChem | |
URL | https://pubchem.ncbi.nlm.nih.gov | |
Description | Data deposited in or computed by PubChem | |
Molecular Formula |
C12H15NO2 | |
Source | PubChem | |
URL | https://pubchem.ncbi.nlm.nih.gov | |
Description | Data deposited in or computed by PubChem | |
Related CAS |
30999-33-8 | |
Record name | 2-Oxiranemethanamine, N-(2-oxiranylmethyl)-N-phenyl-, homopolymer | |
Source | CAS Common Chemistry | |
URL | https://commonchemistry.cas.org/detail?cas_rn=30999-33-8 | |
Description | CAS Common Chemistry is an open community resource for accessing chemical information. Nearly 500,000 chemical substances from CAS REGISTRY cover areas of community interest, including common and frequently regulated chemicals, and those relevant to high school and undergraduate chemistry classes. This chemical information, curated by our expert scientists, is provided in alignment with our mission as a division of the American Chemical Society. | |
Explanation | The data from CAS Common Chemistry is provided under a CC-BY-NC 4.0 license, unless otherwise stated. | |
DSSTOX Substance ID |
DTXSID50862832 | |
Record name | 2-Oxiranemethanamine, N-(2-oxiranylmethyl)-N-phenyl- | |
Source | EPA DSSTox | |
URL | https://comptox.epa.gov/dashboard/DTXSID50862832 | |
Description | DSSTox provides a high quality public chemistry resource for supporting improved predictive toxicology. | |
Molecular Weight |
205.25 g/mol | |
Source | PubChem | |
URL | https://pubchem.ncbi.nlm.nih.gov | |
Description | Data deposited in or computed by PubChem | |
CAS No. |
2095-06-9, 32144-31-3 | |
Record name | N,N-Diglycidylaniline | |
Source | CAS Common Chemistry | |
URL | https://commonchemistry.cas.org/detail?cas_rn=2095-06-9 | |
Description | CAS Common Chemistry is an open community resource for accessing chemical information. Nearly 500,000 chemical substances from CAS REGISTRY cover areas of community interest, including common and frequently regulated chemicals, and those relevant to high school and undergraduate chemistry classes. This chemical information, curated by our expert scientists, is provided in alignment with our mission as a division of the American Chemical Society. | |
Explanation | The data from CAS Common Chemistry is provided under a CC-BY-NC 4.0 license, unless otherwise stated. | |
Record name | Diglycidylaniline | |
Source | ChemIDplus | |
URL | https://pubchem.ncbi.nlm.nih.gov/substance/?source=chemidplus&sourceid=0002095069 | |
Description | ChemIDplus is a free, web search system that provides access to the structure and nomenclature authority files used for the identification of chemical substances cited in National Library of Medicine (NLM) databases, including the TOXNET system. | |
Record name | Aniline, bis(2,3-epoxypropyl)- | |
Source | ChemIDplus | |
URL | https://pubchem.ncbi.nlm.nih.gov/substance/?source=chemidplus&sourceid=0032144313 | |
Description | ChemIDplus is a free, web search system that provides access to the structure and nomenclature authority files used for the identification of chemical substances cited in National Library of Medicine (NLM) databases, including the TOXNET system. | |
Record name | N,N-Diglycidylaniline | |
Source | DTP/NCI | |
URL | https://dtp.cancer.gov/dtpstandard/servlet/dwindex?searchtype=NSC&outputformat=html&searchlist=35364 | |
Description | The NCI Development Therapeutics Program (DTP) provides services and resources to the academic and private-sector research communities worldwide to facilitate the discovery and development of new cancer therapeutic agents. | |
Explanation | Unless otherwise indicated, all text within NCI products is free of copyright and may be reused without our permission. Credit the National Cancer Institute as the source. | |
Record name | 2-Oxiranemethanamine, N-(2-oxiranylmethyl)-N-phenyl- | |
Source | EPA Chemicals under the TSCA | |
URL | https://www.epa.gov/chemicals-under-tsca | |
Description | EPA Chemicals under the Toxic Substances Control Act (TSCA) collection contains information on chemicals and their regulations under TSCA, including non-confidential content from the TSCA Chemical Substance Inventory and Chemical Data Reporting. | |
Record name | 2-Oxiranemethanamine, N-(2-oxiranylmethyl)-N-phenyl- | |
Source | EPA DSSTox | |
URL | https://comptox.epa.gov/dashboard/DTXSID50862832 | |
Description | DSSTox provides a high quality public chemistry resource for supporting improved predictive toxicology. | |
Record name | N,N-bis(2,3-epoxypropyl)aniline | |
Source | European Chemicals Agency (ECHA) | |
URL | https://echa.europa.eu/substance-information/-/substanceinfo/100.016.599 | |
Description | The European Chemicals Agency (ECHA) is an agency of the European Union which is the driving force among regulatory authorities in implementing the EU's groundbreaking chemicals legislation for the benefit of human health and the environment as well as for innovation and competitiveness. | |
Explanation | Use of the information, documents and data from the ECHA website is subject to the terms and conditions of this Legal Notice, and subject to other binding limitations provided for under applicable law, the information, documents and data made available on the ECHA website may be reproduced, distributed and/or used, totally or in part, for non-commercial purposes provided that ECHA is acknowledged as the source: "Source: European Chemicals Agency, http://echa.europa.eu/". Such acknowledgement must be included in each copy of the material. ECHA permits and encourages organisations and individuals to create links to the ECHA website under the following cumulative conditions: Links can only be made to webpages that provide a link to the Legal Notice page. | |
Record name | DIGLYCIDYLANILINE | |
Source | FDA Global Substance Registration System (GSRS) | |
URL | https://gsrs.ncats.nih.gov/ginas/app/beta/substances/AZG5ZPK0BD | |
Description | The FDA Global Substance Registration System (GSRS) enables the efficient and accurate exchange of information on what substances are in regulated products. Instead of relying on names, which vary across regulatory domains, countries, and regions, the GSRS knowledge base makes it possible for substances to be defined by standardized, scientific descriptions. | |
Explanation | Unless otherwise noted, the contents of the FDA website (www.fda.gov), both text and graphics, are not copyrighted. They are in the public domain and may be republished, reprinted and otherwise used freely by anyone without the need to obtain permission from FDA. Credit to the U.S. Food and Drug Administration as the source is appreciated but not required. | |
Retrosynthesis Analysis
AI-Powered Synthesis Planning: Our tool employs the Template_relevance Pistachio, Template_relevance Bkms_metabolic, Template_relevance Pistachio_ringbreaker, Template_relevance Reaxys, Template_relevance Reaxys_biocatalysis model, leveraging a vast database of chemical reactions to predict feasible synthetic routes.
One-Step Synthesis Focus: Specifically designed for one-step synthesis, it provides concise and direct routes for your target compounds, streamlining the synthesis process.
Accurate Predictions: Utilizing the extensive PISTACHIO, BKMS_METABOLIC, PISTACHIO_RINGBREAKER, REAXYS, REAXYS_BIOCATALYSIS database, our tool offers high-accuracy predictions, reflecting the latest in chemical research and data.
Strategy Settings
Precursor scoring | Relevance Heuristic |
---|---|
Min. plausibility | 0.01 |
Model | Template_relevance |
Template Set | Pistachio/Bkms_metabolic/Pistachio_ringbreaker/Reaxys/Reaxys_biocatalysis |
Top-N result to add to graph | 6 |
Feasible Synthetic Routes
ANone: Diglycidylaniline has the molecular formula C12H13NO2 and a molecular weight of 203.24 g/mol.
A: Researchers commonly employ techniques like Gas Chromatography (GC) [, ], High-Performance Liquid Chromatography (HPLC) [, , , ], Infrared Spectroscopy (IR) [, ], Nuclear Magnetic Resonance (NMR) spectroscopy (both 1H and 13C) [, , , , , , , ], and Mass Spectroscopy (MS) [, ] to analyze this compound, identify impurities, and study reaction mechanisms.
A: this compound, being bifunctional, tends to form networks with lower glass transition temperatures (Tg) and thermal stability compared to trifunctional epoxy resins like N,N-diglycidyl-4-glycidyloxyaniline (DGOA) when cured with the same tetrafunctional curing agent []. This difference arises from the lower crosslinking density achievable with a bifunctional resin.
A: this compound serves as a key component in epoxy resin formulations. When cured, these formulations exhibit desirable properties like high strength, excellent adhesion, and good chemical resistance, making them suitable for applications in coatings, adhesives, composites, and electronics [, , , , ].
A: Incorporating modified zinc into epoxy coatings formulated with this compound significantly improves their anticorrosion performance on copper substrates [, ]. The optimal zinc content for enhanced corrosion protection was found to be 5 wt% [].
A: The curing process of this compound with aromatic amines is significantly influenced by intramolecular cyclization reactions [, , , , ]. This leads to the formation of small cyclic structures, resulting in networks with lower crosslinking density than expected based on reactant functionality [, ].
A: Higher curing temperatures promote cyclization reactions during the curing of this compound with amines []. This tendency towards cyclization at elevated temperatures results in a reduction in the number-average molecular weight (Mn) of the resulting polymers [].
A: Researchers utilize Density Functional Theory (DFT) calculations to optimize the geometry of this compound and understand its electronic structure []. These calculations provide insights into the molecule's reactivity and its interactions with other molecules during the curing process.
A: Using a mixture of tetrahydrofuran (THF) and dioxane as a solvent for spin-casting films of aniline-based azopolymers, which incorporate this compound, results in improved diffraction efficiency compared to films cast from THF alone []. This enhancement is attributed to the residual dioxane in the film, which creates a larger free volume in the solid state, benefiting optical properties.
A: Researchers are exploring the use of circular economy materials, such as hydroxyapatite extracted from biological waste, to develop flame-retardant epoxy composites []. Combining hydroxyapatite with this compound and other flame-retardant components shows promise in enhancing the fire safety of these materials while promoting resource efficiency and waste reduction.
Disclaimer and Information on In-Vitro Research Products
Please be aware that all articles and product information presented on BenchChem are intended solely for informational purposes. The products available for purchase on BenchChem are specifically designed for in-vitro studies, which are conducted outside of living organisms. In-vitro studies, derived from the Latin term "in glass," involve experiments performed in controlled laboratory settings using cells or tissues. It is important to note that these products are not categorized as medicines or drugs, and they have not received approval from the FDA for the prevention, treatment, or cure of any medical condition, ailment, or disease. We must emphasize that any form of bodily introduction of these products into humans or animals is strictly prohibited by law. It is essential to adhere to these guidelines to ensure compliance with legal and ethical standards in research and experimentation.