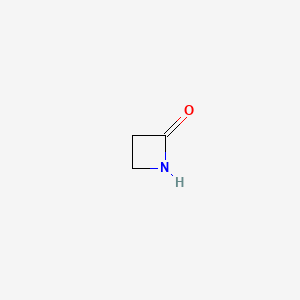
azetidin-2-one
Overview
Description
Mechanism of Action
Target of Action
Azetidin-2-one has been found to exhibit diversified biological and pharmacological activity . It has been identified as a potential inhibitor of human HDAC6 and HDAC8 enzymes . These enzymes are involved in the regulation of gene expression and protein function, making them important targets for therapeutic intervention.
Biochemical Analysis
Biochemical Properties
2-Azetidinone plays a crucial role in biochemical reactions, particularly in the inhibition of bacterial cell wall biosynthesis. It interacts with penicillin-binding proteins (PBPs), which are essential enzymes in the synthesis of peptidoglycan, a major component of the bacterial cell wall. By mimicking the D-Ala-D-Ala substrate of PBPs, 2-Azetidinone forms a covalent bond with the active site serine residue of these enzymes, thereby inhibiting their activity and preventing cell wall formation . This interaction is critical for the antibacterial properties of beta-lactam antibiotics.
Cellular Effects
2-Azetidinone exerts significant effects on various types of cells and cellular processes. In bacterial cells, it disrupts cell wall synthesis, leading to cell lysis and death. This compound also influences cell signaling pathways and gene expression by inhibiting the synthesis of peptidoglycan, which is essential for maintaining cell shape and integrity . In mammalian cells, derivatives of 2-Azetidinone, such as ezetimibe, have been shown to inhibit cholesterol absorption in the intestine, thereby affecting lipid metabolism and reducing plasma cholesterol levels .
Molecular Mechanism
The molecular mechanism of 2-Azetidinone involves its ability to bind to and inhibit the activity of PBPs. This binding prevents the cross-linking of peptidoglycan strands, which is essential for bacterial cell wall integrity. Additionally, 2-Azetidinone derivatives, such as ezetimibe, inhibit the Niemann-Pick C1-Like 1 (NPC1L1) protein in the intestine, reducing cholesterol absorption . This inhibition is achieved through noncovalent interactions with the protein, leading to decreased cholesterol levels in the bloodstream .
Temporal Effects in Laboratory Settings
In laboratory settings, the effects of 2-Azetidinone can vary over time. The compound is hydrolytically vulnerable and can degrade under certain conditions, affecting its stability and efficacy . Long-term studies have shown that 2-Azetidinone and its derivatives can maintain their biological activity over extended periods, although their stability may be influenced by factors such as temperature and pH . In in vitro and in vivo studies, the compound has demonstrated sustained antibacterial and cholesterol-lowering effects .
Dosage Effects in Animal Models
The effects of 2-Azetidinone vary with different dosages in animal models. At therapeutic doses, the compound effectively inhibits bacterial cell wall synthesis and reduces cholesterol absorption without causing significant adverse effects . At higher doses, 2-Azetidinone can exhibit toxic effects, including liver function abnormalities and muscle damage . These threshold effects highlight the importance of optimizing dosage to achieve the desired therapeutic outcomes while minimizing toxicity.
Metabolic Pathways
2-Azetidinone is involved in several metabolic pathways, particularly those related to cholesterol metabolism. Ezetimibe, a derivative of 2-Azetidinone, inhibits the NPC1L1 protein, reducing the absorption of dietary and biliary cholesterol in the intestine . This inhibition leads to increased uptake of low-density lipoprotein (LDL) cholesterol by the liver, thereby lowering plasma cholesterol levels . Additionally, 2-Azetidinone derivatives can affect metabolic flux and metabolite levels by modulating enzyme activity and gene expression .
Transport and Distribution
The transport and distribution of 2-Azetidinone within cells and tissues are mediated by specific transporters and binding proteins. Ezetimibe, for example, is absorbed in the intestine and transported to the liver, where it exerts its cholesterol-lowering effects . The compound and its metabolites are distributed to various tissues, including the liver, intestine, and plasma . The localization and accumulation of 2-Azetidinone are influenced by factors such as lipid solubility and protein binding .
Subcellular Localization
The subcellular localization of 2-Azetidinone and its derivatives is critical for their activity and function. In bacterial cells, 2-Azetidinone targets the cell wall synthesis machinery, localizing to the periplasmic space where PBPs are found . In mammalian cells, ezetimibe localizes to the brush border membrane of enterocytes in the small intestine, where it inhibits cholesterol absorption . The targeting of 2-Azetidinone to specific cellular compartments is facilitated by post-translational modifications and protein-protein interactions .
Preparation Methods
Synthetic Routes and Reaction Conditions
Azetidin-2-one can be synthesized through several methods. This reaction typically involves the use of acyl halides and tertiary amines to generate ketenes, which then react with imines to form the this compound ring . Another method involves the carbonylation of acyclic diaminocarbenes, leading to diaminoketenes, which undergo a retro-Wolff rearrangement followed by an intramolecular C–H insertion .
Industrial Production Methods
Industrial production of this compound often employs green chemistry principles to enhance efficiency and reduce environmental impact. For instance, the use of propylphosphonic anhydride (T3P®) as an acid activator in the [2+2] ketene-imine cycloaddition has been shown to yield high-purity products under mild conditions .
Chemical Reactions Analysis
Types of Reactions
Azetidin-2-one undergoes various chemical reactions, including oxidation, reduction, and substitution . The ring strain in the four-membered structure makes it particularly reactive under certain conditions .
Common Reagents and Conditions
Oxidation: This compound can be oxidized using reagents like ceric ammonium nitrate.
Reduction: Reduction reactions often involve hydrogenation or the use of metal hydrides.
Substitution: Substitution reactions can be facilitated by reagents such as alkyl halides and bases.
Major Products
The major products formed from these reactions include various substituted azetidin-2-ones, which can exhibit different biological activities .
Scientific Research Applications
Azetidin-2-one has a wide range of applications in scientific research:
Comparison with Similar Compounds
Similar Compounds
Aziridines: Three-membered nitrogen-containing rings that are more strained and reactive than azetidin-2-one.
Pyrrolidines: Five-membered nitrogen-containing rings that are less strained and exhibit different reactivity patterns.
Indolones: Compounds with a fused ring system that exhibit unique biological activities.
Uniqueness
This compound’s unique four-membered ring structure imparts specific reactivity and biological properties that are distinct from other nitrogen-containing heterocycles . Its ability to form stable β-lactam antibiotics highlights its significance in medicinal chemistry .
Biological Activity
Azetidin-2-one, also known as β-lactam, is a cyclic amide with significant biological activity, particularly in the fields of medicinal chemistry and pharmacology. This article provides a detailed examination of the biological activities associated with this compound derivatives, focusing on their anticancer, anti-inflammatory, and antimicrobial properties.
The this compound structure is characterized by a four-membered ring containing a nitrogen atom and a carbonyl group. This unique configuration allows for diverse interactions with biological targets, particularly proteins involved in cell cycle regulation and apoptosis.
Research indicates that certain this compound derivatives exhibit potent anticancer activity. For instance, a study evaluating 16 derivatives demonstrated that compound 6 (N-(p-methoxy-phenyl)-2-(p-methyl-phenyl)-3-phenoxy-azetidin-2-one) induced apoptosis in SiHa and B16F10 cancer cell lines. The mechanism involves the inhibition of specific cell cycle genes and the induction of caspase-3 activity, leading to programmed cell death .
Anticancer Activity
The anticancer potential of this compound derivatives has been extensively studied. The following table summarizes the apoptotic activity of selected compounds compared to podophyllotoxin, a known anticancer agent:
Compound | Apoptotic Activity (%) |
---|---|
Podophyllotoxin | 100% |
Compound 6 | 100% |
Compound 8 | 88% |
Compound 14 | 93% |
Negative Control (untreated cells) | 0% |
These findings suggest that this compound derivatives can effectively induce apoptosis in cancer cells, making them promising candidates for further development as anticancer drugs .
Anti-inflammatory Properties
In addition to their anticancer effects, this compound derivatives have shown significant anti-inflammatory activity. A study on this compound derivatives of ferulic acid revealed their effectiveness in models of acute and chronic inflammation:
- Acute Inflammation : The compounds exhibited maximum anti-inflammatory effects 24 hours post-administration in carrageenan-induced inflammation models.
- Chronic Inflammation : In granuloma tests, the most active compound was identified as compound 6b , which demonstrated comparable effects to established anti-inflammatory drugs like diclofenac sodium .
Liver Function Assessment
The safety profile of these compounds was evaluated through serum liver enzyme assays (AST, ALT, LDH), showing no significant adverse effects compared to healthy controls. Histopathological examinations of liver, lung, and kidney tissues revealed no major changes in treated groups .
Antimicrobial Activity
This compound derivatives have also been investigated for their antimicrobial properties. Research indicates that several compounds exhibit significant antibacterial and antifungal activities. For instance, some derivatives have shown promising results against Mycobacterium tuberculosis .
Case Studies and Research Findings
- Caspase Activation : A study highlighted that this compound compounds induce apoptosis through caspase activation, confirming their role in programmed cell death mechanisms .
- Microtubule Interaction : The interaction of this compound derivatives with tubulin at the colchicine binding site suggests a mechanism similar to that of other microtubule-targeting agents used in cancer therapy .
- Toxicity Studies : In vivo studies on acute toxicity revealed that while some compounds possess moderate toxicity levels, they are generally well-tolerated compared to traditional chemotherapeutics .
Properties
IUPAC Name |
azetidin-2-one | |
---|---|---|
Source | PubChem | |
URL | https://pubchem.ncbi.nlm.nih.gov | |
Description | Data deposited in or computed by PubChem | |
InChI |
InChI=1S/C3H5NO/c5-3-1-2-4-3/h1-2H2,(H,4,5) | |
Source | PubChem | |
URL | https://pubchem.ncbi.nlm.nih.gov | |
Description | Data deposited in or computed by PubChem | |
InChI Key |
MNFORVFSTILPAW-UHFFFAOYSA-N | |
Source | PubChem | |
URL | https://pubchem.ncbi.nlm.nih.gov | |
Description | Data deposited in or computed by PubChem | |
Canonical SMILES |
C1CNC1=O | |
Source | PubChem | |
URL | https://pubchem.ncbi.nlm.nih.gov | |
Description | Data deposited in or computed by PubChem | |
Molecular Formula |
C3H5NO | |
Source | PubChem | |
URL | https://pubchem.ncbi.nlm.nih.gov | |
Description | Data deposited in or computed by PubChem | |
DSSTOX Substance ID |
DTXSID30239225 | |
Record name | 2-Azetidinone | |
Source | EPA DSSTox | |
URL | https://comptox.epa.gov/dashboard/DTXSID30239225 | |
Description | DSSTox provides a high quality public chemistry resource for supporting improved predictive toxicology. | |
Molecular Weight |
71.08 g/mol | |
Source | PubChem | |
URL | https://pubchem.ncbi.nlm.nih.gov | |
Description | Data deposited in or computed by PubChem | |
Physical Description |
Beige crystalline solid; [Sigma-Aldrich MSDS] | |
Record name | 2-Azetidinone | |
Source | Haz-Map, Information on Hazardous Chemicals and Occupational Diseases | |
URL | https://haz-map.com/Agents/19524 | |
Description | Haz-Map® is an occupational health database designed for health and safety professionals and for consumers seeking information about the adverse effects of workplace exposures to chemical and biological agents. | |
Explanation | Copyright (c) 2022 Haz-Map(R). All rights reserved. Unless otherwise indicated, all materials from Haz-Map are copyrighted by Haz-Map(R). No part of these materials, either text or image may be used for any purpose other than for personal use. Therefore, reproduction, modification, storage in a retrieval system or retransmission, in any form or by any means, electronic, mechanical or otherwise, for reasons other than personal use, is strictly prohibited without prior written permission. | |
CAS No. |
930-21-2 | |
Record name | 2-Azetidinone | |
Source | CAS Common Chemistry | |
URL | https://commonchemistry.cas.org/detail?cas_rn=930-21-2 | |
Description | CAS Common Chemistry is an open community resource for accessing chemical information. Nearly 500,000 chemical substances from CAS REGISTRY cover areas of community interest, including common and frequently regulated chemicals, and those relevant to high school and undergraduate chemistry classes. This chemical information, curated by our expert scientists, is provided in alignment with our mission as a division of the American Chemical Society. | |
Explanation | The data from CAS Common Chemistry is provided under a CC-BY-NC 4.0 license, unless otherwise stated. | |
Record name | 2-Azetidinone | |
Source | ChemIDplus | |
URL | https://pubchem.ncbi.nlm.nih.gov/substance/?source=chemidplus&sourceid=0000930212 | |
Description | ChemIDplus is a free, web search system that provides access to the structure and nomenclature authority files used for the identification of chemical substances cited in National Library of Medicine (NLM) databases, including the TOXNET system. | |
Record name | 2-Azetidinone | |
Source | EPA DSSTox | |
URL | https://comptox.epa.gov/dashboard/DTXSID30239225 | |
Description | DSSTox provides a high quality public chemistry resource for supporting improved predictive toxicology. | |
Record name | azetidin-2-one | |
Source | European Chemicals Agency (ECHA) | |
URL | https://echa.europa.eu/information-on-chemicals | |
Description | The European Chemicals Agency (ECHA) is an agency of the European Union which is the driving force among regulatory authorities in implementing the EU's groundbreaking chemicals legislation for the benefit of human health and the environment as well as for innovation and competitiveness. | |
Explanation | Use of the information, documents and data from the ECHA website is subject to the terms and conditions of this Legal Notice, and subject to other binding limitations provided for under applicable law, the information, documents and data made available on the ECHA website may be reproduced, distributed and/or used, totally or in part, for non-commercial purposes provided that ECHA is acknowledged as the source: "Source: European Chemicals Agency, http://echa.europa.eu/". Such acknowledgement must be included in each copy of the material. ECHA permits and encourages organisations and individuals to create links to the ECHA website under the following cumulative conditions: Links can only be made to webpages that provide a link to the Legal Notice page. | |
Synthesis routes and methods
Procedure details
Retrosynthesis Analysis
AI-Powered Synthesis Planning: Our tool employs the Template_relevance Pistachio, Template_relevance Bkms_metabolic, Template_relevance Pistachio_ringbreaker, Template_relevance Reaxys, Template_relevance Reaxys_biocatalysis model, leveraging a vast database of chemical reactions to predict feasible synthetic routes.
One-Step Synthesis Focus: Specifically designed for one-step synthesis, it provides concise and direct routes for your target compounds, streamlining the synthesis process.
Accurate Predictions: Utilizing the extensive PISTACHIO, BKMS_METABOLIC, PISTACHIO_RINGBREAKER, REAXYS, REAXYS_BIOCATALYSIS database, our tool offers high-accuracy predictions, reflecting the latest in chemical research and data.
Strategy Settings
Precursor scoring | Relevance Heuristic |
---|---|
Min. plausibility | 0.01 |
Model | Template_relevance |
Template Set | Pistachio/Bkms_metabolic/Pistachio_ringbreaker/Reaxys/Reaxys_biocatalysis |
Top-N result to add to graph | 6 |
Feasible Synthetic Routes
Q1: What is the molecular formula and weight of 2-azetidinone?
A1: The molecular formula of 2-azetidinone is C3H5NO, and its molecular weight is 71.08 g/mol.
Q2: How can 2-azetidinones be characterized spectroscopically?
A2: 2-Azetidinones can be characterized using various spectroscopic techniques, including:
Q3: What is the most common method for synthesizing 2-azetidinones?
A3: The Staudinger ketene-imine cycloaddition reaction [2+2] is the most widely employed method for synthesizing 2-azetidinones []. This reaction involves the reaction of a ketene, generated in situ from an acid chloride, with an imine to form the β-lactam ring.
Q4: Can you describe a greener approach to 2-azetidinone synthesis?
A4: Researchers are exploring greener methods for 2-azetidinone synthesis. One such approach utilizes microwave irradiation and catalytic amounts of molecular iodine, offering a more sustainable alternative [].
Q5: How can the Pauson-Khand reaction be used in the synthesis of 2-azetidinones?
A5: The Pauson-Khand reaction can be employed to synthesize fused tricyclic 2-azetidinones []. This reaction involves the [2+2+1] cycloaddition of an alkyne, an alkene, and carbon monoxide in the presence of a cobalt catalyst. By tethering an enyne system to a 2-azetidinone ring, the Pauson-Khand reaction can be used to create complex tricyclic structures.
Q6: How can ring-closing metathesis (RCM) be used in 2-azetidinone chemistry?
A6: RCM, employing catalysts like Grubbs' carbene, enables the formation of medium-sized rings fused to 2-azetidinone systems [, ]. This approach allows the creation of structurally diverse 2-azetidinones, including bicyclic and tricyclic systems, by tethering appropriate alkene moieties to the β-lactam ring and then performing RCM.
Q7: What is the significance of 4-substituted 2-azetidinones?
A7: 4-Substituted 2-azetidinones, particularly those with a heteroatom at C4, are significant as they can act as precursors to novel bicyclic β-lactams []. The introduction of alkyl groups at the C4 position can enhance activity against Gram-negative bacteria and increase stability against β-lactamases.
Q8: What interesting rearrangements can 2-azetidinones undergo?
A8: 2-Azetidinones can undergo several interesting rearrangements, including:
- Ring expansion: 4-(1-haloalkyl)- and 4-(2-haloalkyl)-2-azetidinones can undergo ring expansion reactions to yield γ-lactams (2-pyrrolidinones). This transformation occurs via transient aziridine or azetidine intermediates and offers a route to highly functionalized γ-lactams [, ].
- Isomerization: cis-4-Formyl-2-azetidinones can undergo base-promoted isomerization to their corresponding trans isomers. This transformation is valuable for accessing specific diastereomers of 4-formyl-2-azetidinones, which are useful synthetic intermediates [].
- Rearrangement to Cyclic Enaminones: Under certain basic conditions, cis-4-formyl-2-azetidinones can rearrange to form cyclic enaminones. This rearrangement proceeds through an E1cB elimination mechanism and provides access to a different class of heterocyclic compounds [].
Q9: Why are 2-azetidinones important in medicinal chemistry?
A9: 2-Azetidinones form the core structure of various important antibiotics, including penicillins, cephalosporins, and carbapenems [, ]. These antibiotics exert their biological activity by inhibiting bacterial cell wall synthesis.
Q10: What other biological activities have been reported for 2-azetidinones?
A10: Apart from their antibacterial activity, 2-azetidinones have shown promise in a range of other therapeutic areas, including:
- Cholesterol absorption inhibitors: Certain 1,3,4-trisubstituted-β-lactams have demonstrated potent cholesterol absorption inhibitory activity, making them potential drug candidates for treating hypercholesterolemia [].
- Human cytomegalovirus (HCMV) protease inhibitors: Some 2-azetidinones have exhibited inhibitory activity against HCMV protease, a target for antiviral therapy [].
- Thrombin inhibitors: Specific 2-azetidinone derivatives have shown inhibitory activity against thrombin, a key enzyme involved in blood coagulation, suggesting their potential as anticoagulant agents [].
Q11: Can you provide an example of a 2-azetidinone with potent antiproliferative activity?
A11: Compound 10n (3-chloro-4-(3-hydroxy-4-methoxy-phenyl)-1-(3,4,5-trimethoxyphenyl)azetidin-2-one) exhibited potent antiproliferative activity against MCF-7 breast cancer cells, with an IC50 value of 17 nM. This compound targets tubulin, a protein involved in cell division, and causes mitotic catastrophe, leading to cell death [].
Q12: How do structural modifications on the 2-azetidinone ring impact its biological activity?
A12: Structural modifications of the 2-azetidinone ring significantly influence its biological activity, potency, and selectivity. For example:
- Substituents at C3 and C4: Introducing various substituents at the C3 and C4 positions can alter the compound's interaction with its biological target, potentially improving its activity or selectivity [, ].
- N1 Substituents: Modifications at the N1 position can affect the compound's pharmacokinetic properties, such as its absorption, distribution, metabolism, and excretion (ADME), ultimately influencing its efficacy and duration of action [].
- Stereochemistry: The stereochemistry of the substituents around the β-lactam ring plays a crucial role in determining the compound's biological activity. Often, specific stereoisomers exhibit significantly higher potency and selectivity compared to others [].
Q13: What are the challenges associated with developing 2-azetidinones as therapeutics?
A13: While 2-azetidinones hold significant promise as therapeutic agents, several challenges need to be addressed:
- Drug resistance: The emergence of bacterial resistance to β-lactam antibiotics is a major concern. Understanding the mechanisms of resistance and developing strategies to overcome them is crucial [].
- Stability and formulation: Some 2-azetidinones can be chemically unstable or poorly soluble, making formulation and delivery challenging []. Research efforts focus on developing stable formulations and exploring novel drug delivery systems to improve their bioavailability and therapeutic efficacy [].
- Toxicity and safety: As with any drug candidate, thoroughly evaluating the toxicity and safety profile of 2-azetidinones is paramount []. This involves assessing acute and chronic toxicity, potential for drug interactions, and long-term effects.
Q14: What are the future directions in 2-azetidinone research?
A14: Research on 2-azetidinones continues to flourish, driven by their therapeutic potential and synthetic versatility. Some key areas of future exploration include:
- Developing new synthetic methods: Exploring more efficient, enantioselective, and environmentally friendly synthetic routes to access structurally diverse 2-azetidinones is essential [].
- Expanding the therapeutic scope: Investigating the potential of 2-azetidinones beyond their established use as antibiotics and exploring their application in other areas like antivirals, anticancer agents, and enzyme inhibitors [].
- Understanding the structure-activity relationships (SAR): Delineating the precise SAR governing the biological activity of 2-azetidinones is crucial for designing more potent and selective drug candidates []. This involves systematic modifications of the β-lactam ring and evaluating their impact on activity, potency, and selectivity.
- Exploring novel drug delivery strategies: Developing innovative drug delivery systems, such as nanoparticles or targeted delivery vehicles, can enhance the bioavailability and therapeutic index of 2-azetidinones, improving their efficacy and reducing potential side effects [].
Disclaimer and Information on In-Vitro Research Products
Please be aware that all articles and product information presented on BenchChem are intended solely for informational purposes. The products available for purchase on BenchChem are specifically designed for in-vitro studies, which are conducted outside of living organisms. In-vitro studies, derived from the Latin term "in glass," involve experiments performed in controlled laboratory settings using cells or tissues. It is important to note that these products are not categorized as medicines or drugs, and they have not received approval from the FDA for the prevention, treatment, or cure of any medical condition, ailment, or disease. We must emphasize that any form of bodily introduction of these products into humans or animals is strictly prohibited by law. It is essential to adhere to these guidelines to ensure compliance with legal and ethical standards in research and experimentation.