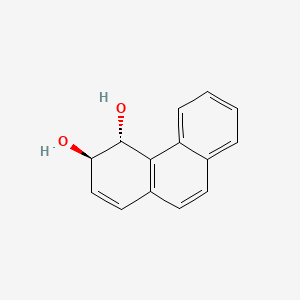
(3R,4R)-3,4-dihydrophenanthrene-3,4-diol
- Click on QUICK INQUIRY to receive a quote from our team of experts.
- With the quality product at a COMPETITIVE price, you can focus more on your research.
Overview
Description
(3R,4R)-3,4-dihydrophenanthrene-3,4-diol is a trans-3,4-dihydrophenanthrene-3,4-diol. It is an enantiomer of a (3S,4S)-3,4-dihydrophenanthrene-3,4-diol.
Scientific Research Applications
Chemical Properties and Structure
(3R,4R)-3,4-dihydrophenanthrene-3,4-diol is a polycyclic aromatic compound characterized by two hydroxyl groups on a phenanthrene backbone. Its unique stereochemistry allows it to participate in various biological and chemical processes. The compound exists as an enantiomer to (3S,4S)-3,4-dihydrophenanthrene-3,4-diol, which has been studied for its metabolic pathways and biological interactions.
Environmental Science
The compound plays a crucial role in the biotransformation of polycyclic aromatic hydrocarbons (PAHs), such as phenanthrene. It is involved in microbial degradation processes that help in the detoxification of environmental pollutants. Research indicates that this compound can serve as a biomarker for PAH exposure and metabolism in humans. For instance, studies have quantified dihydrodiol metabolites in the urine of smokers to understand the impact of PAHs on human health .
Application Area | Key Findings |
---|---|
Biomarker for PAH | Detected in urine samples; indicates exposure to phenanthrene and other PAHs. |
Microbial Degradation | Participates in enzymatic reactions that convert PAHs into less harmful substances. |
Pharmacology
In pharmacological research, this compound has shown potential as a candidate for drug development due to its biological activity. Studies have indicated that it may influence oxidative stress pathways and contribute to understanding carcinogenic processes associated with PAHs .
Pharmacological Aspect | Observations |
---|---|
Oxidative Stress | May interact with biological systems affecting oxidative stress pathways. |
Carcinogenicity | Investigated for its role in the metabolism of carcinogenic compounds. |
Nanotechnology
Recent advancements have highlighted the use of polyphenol-containing nanoparticles for biomedical applications. This compound could be integrated into nanoparticle formulations due to its structural properties and biological activity. These nanoparticles have shown promise in drug delivery systems and therapeutic applications due to their ability to interact with biological molecules effectively .
Nanotechnology Application | Benefits |
---|---|
Drug Delivery Systems | Enhances bioavailability and targeted delivery of therapeutic agents. |
Therapeutic Applications | Potential use in cancer therapy and bioimaging due to unique properties. |
Case Study 1: Urinary Biomarkers in Smokers
A study conducted on smokers quantified dihydrodiol metabolites of phenanthrene in urine samples. The results indicated significant differences in metabolite ratios between smokers and non-smokers, suggesting that this compound could serve as an effective biomarker for exposure assessment .
Case Study 2: Polyphenol Nanoparticles
Research into polyphenol-containing nanoparticles demonstrated their versatility in biomedical applications. The integration of compounds like this compound into these nanoparticles could enhance their therapeutic efficacy against cancer cells while minimizing toxicity .
Properties
CAS No. |
77123-17-2 |
---|---|
Molecular Formula |
C14H12O2 |
Molecular Weight |
212.24 g/mol |
IUPAC Name |
(3R,4R)-3,4-dihydrophenanthrene-3,4-diol |
InChI |
InChI=1S/C14H12O2/c15-12-8-7-10-6-5-9-3-1-2-4-11(9)13(10)14(12)16/h1-8,12,14-16H/t12-,14+/m1/s1 |
InChI Key |
FOTICWSJABVKPW-OCCSQVGLSA-N |
SMILES |
C1=CC=C2C(=C1)C=CC3=C2C(C(C=C3)O)O |
Isomeric SMILES |
C1=CC=C2C(=C1)C=CC3=C2[C@H]([C@@H](C=C3)O)O |
Canonical SMILES |
C1=CC=C2C(=C1)C=CC3=C2C(C(C=C3)O)O |
Key on ui other cas no. |
77123-17-2 |
Origin of Product |
United States |
Retrosynthesis Analysis
AI-Powered Synthesis Planning: Our tool employs the Template_relevance Pistachio, Template_relevance Bkms_metabolic, Template_relevance Pistachio_ringbreaker, Template_relevance Reaxys, Template_relevance Reaxys_biocatalysis model, leveraging a vast database of chemical reactions to predict feasible synthetic routes.
One-Step Synthesis Focus: Specifically designed for one-step synthesis, it provides concise and direct routes for your target compounds, streamlining the synthesis process.
Accurate Predictions: Utilizing the extensive PISTACHIO, BKMS_METABOLIC, PISTACHIO_RINGBREAKER, REAXYS, REAXYS_BIOCATALYSIS database, our tool offers high-accuracy predictions, reflecting the latest in chemical research and data.
Strategy Settings
Precursor scoring | Relevance Heuristic |
---|---|
Min. plausibility | 0.01 |
Model | Template_relevance |
Template Set | Pistachio/Bkms_metabolic/Pistachio_ringbreaker/Reaxys/Reaxys_biocatalysis |
Top-N result to add to graph | 6 |
Feasible Synthetic Routes
Disclaimer and Information on In-Vitro Research Products
Please be aware that all articles and product information presented on BenchChem are intended solely for informational purposes. The products available for purchase on BenchChem are specifically designed for in-vitro studies, which are conducted outside of living organisms. In-vitro studies, derived from the Latin term "in glass," involve experiments performed in controlled laboratory settings using cells or tissues. It is important to note that these products are not categorized as medicines or drugs, and they have not received approval from the FDA for the prevention, treatment, or cure of any medical condition, ailment, or disease. We must emphasize that any form of bodily introduction of these products into humans or animals is strictly prohibited by law. It is essential to adhere to these guidelines to ensure compliance with legal and ethical standards in research and experimentation.