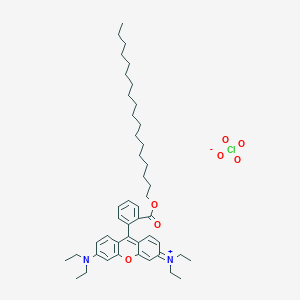
Rhodamine B octadecyl ester perchlorate
Overview
Description
Rhodamine B octadecyl ester perchlorate is a fluorescent dye known for its lipophilic properties, making it suitable for various scientific applications. It is a derivative of Rhodamine B, modified to include an octadecyl ester group, which enhances its solubility in organic solvents and its ability to integrate into lipid environments .
Mechanism of Action
Target of Action
Rhodamine B octadecyl ester perchlorate is a lipophilic energy transfer acceptor . It is selectively localized in mitochondria , making these organelles the primary targets of its action. Mitochondria play a crucial role in energy production, cell signaling, and apoptosis.
Mode of Action
This compound interacts with its targets (mitochondria) by being selectively localized within them . As a lipophilic energy transfer acceptor, it can accept energy from lipophilic fluoresceins . This energy transfer can be used in fluorescence energy transfer assays for cell fusion .
Pharmacokinetics
It is known to be soluble in dmso and dmf , which could potentially impact its bioavailability.
Result of Action
The result of this compound’s action is the transfer of energy from lipophilic fluoresceins . This energy transfer can be used in fluorescence energy transfer assays for cell fusion . Additionally, it has been used as a probe for measuring potassium in fiber-optic sensors .
Action Environment
It is known to be stable under desiccating conditions and can be stored at temperatures between 2-8°c .
Preparation Methods
Synthetic Routes and Reaction Conditions
The synthesis of Rhodamine B octadecyl ester perchlorate typically involves the esterification of Rhodamine B with octadecanol in the presence of a suitable catalyst. The reaction is carried out under reflux conditions in an organic solvent such as dichloromethane. The product is then purified through recrystallization or chromatography to obtain a high-purity compound .
Industrial Production Methods
Industrial production of this compound follows similar synthetic routes but on a larger scale. The process involves the use of industrial-grade solvents and catalysts, with stringent control over reaction conditions to ensure consistent product quality. The final product is subjected to rigorous quality control measures, including purity testing and fluorescence efficiency assessments .
Chemical Reactions Analysis
Types of Reactions
Rhodamine B octadecyl ester perchlorate primarily undergoes substitution reactions due to the presence of reactive functional groups. It can also participate in oxidation and reduction reactions under specific conditions .
Common Reagents and Conditions
Substitution Reactions: Typically involve nucleophiles such as amines or thiols in organic solvents.
Oxidation Reactions: Can be carried out using oxidizing agents like hydrogen peroxide or potassium permanganate.
Reduction Reactions: Often involve reducing agents such as sodium borohydride or lithium aluminum hydride.
Major Products
The major products formed from these reactions depend on the specific reagents and conditions used. For example, substitution reactions with amines can yield amide derivatives, while oxidation reactions can produce various oxidized forms of the dye .
Scientific Research Applications
Rhodamine B octadecyl ester perchlorate is widely used in scientific research due to its unique properties:
Chemistry: Utilized as a fluorescent probe in various chemical assays and reactions.
Biology: Employed in cell imaging and tracking due to its ability to integrate into lipid membranes.
Medicine: Used in drug delivery systems, particularly in the design of nanoparticles for targeted drug delivery.
Industry: Applied in the development of sensors and diagnostic tools, leveraging its fluorescence properties for sensitive detection
Comparison with Similar Compounds
Similar Compounds
Rhodamine B: The parent compound, less lipophilic but widely used in similar applications.
Rhodamine 6G: Another fluorescent dye with different spectral properties, used in laser applications.
Rhodamine 110 chloride: A derivative with distinct fluorescence characteristics, used in biochemical assays
Uniqueness
Rhodamine B octadecyl ester perchlorate stands out due to its enhanced lipophilicity, making it particularly suitable for applications involving lipid environments. Its ability to integrate into lipid membranes and its high fluorescence efficiency make it a valuable tool in various scientific fields .
Biological Activity
Rhodamine B octadecyl ester perchlorate (C18RB) is a derivative of the well-known fluorescent dye Rhodamine B, modified to enhance its hydrophobic properties and biological applications. This compound has garnered attention in various fields, including drug delivery systems, cellular imaging, and biophysical studies due to its unique properties and functionalities.
This compound is characterized by its molecular formula and a molecular weight of approximately 785.5 g/mol. It appears as a green crystalline solid and is soluble in water, with a pH range of 3-4 when dissolved at concentrations of 10 g/L at 20°C .
1. Cellular Uptake and Endosomal Escape
Recent studies have demonstrated that C18RB can be effectively encapsulated within polymeric nanoparticles, allowing for enhanced cellular uptake. For instance, nanoparticles composed of poly(2-(diethylamino)ethyl methacrylate) (PDEAEMA) were used to encapsulate C18RB, achieving an encapsulation efficiency of approximately 30% . The nanoparticles exhibited a pH-responsive behavior that facilitates endosomal escape, crucial for delivering therapeutic agents into the cytosol of target cells.
In vitro experiments using 3T3 fibroblast cells showed that these nanoparticles maintained over 80% cell viability at concentrations up to 5 mg/mL, indicating low cytotoxicity while allowing for effective cellular localization of the dye .
2. Translocation Across Lipid Membranes
The translocation properties of C18RB across lipid bilayers have been studied extensively. Research indicates that the rate of translocation is influenced by the alkyl chain length of the rhodamine derivatives. C18RB demonstrated a significant ability to traverse lipid membranes, which is vital for its application in drug delivery systems . The translocation rate increased with longer hydrocarbon chains, suggesting that C18RB’s octadecyl group enhances its membrane affinity compared to shorter-chain derivatives.
Applications in Drug Delivery
C18RB has been explored as a model compound for drug delivery systems due to its hydrophobic nature and fluorescence properties. The encapsulation within nanoparticles not only protects the dye from degradation but also improves the solubility and bioavailability of hydrophobic drugs. The ability to escape from endosomal compartments further enhances its potential as a carrier for therapeutic agents.
Study on Nanoparticle Formulation
A study focused on formulating dual-component nanoparticles using C18RB highlighted its role as a fluorophore for tracking nanoparticle localization within cells. The nanoparticles were synthesized through nanoprecipitation methods and showed efficient loading capabilities for hydrophobic drugs. The study concluded that C18RB serves as an effective model dye for assessing nanoparticle behavior in biological systems .
Translocation Studies
Another significant study investigated the voltage-dependent translocation of C18RB across lipid membranes. The findings indicated that C18RB could effectively facilitate proton shuttling through membranes, demonstrating its potential utility in biophysical applications and as a tool for studying membrane dynamics .
Summary Table of Biological Activities
Property | Description |
---|---|
Molecular Formula | |
Molecular Weight | 785.5 g/mol |
Solubility | Soluble in water; pH 3-4 (10 g/L) |
Cell Viability | >80% at concentrations up to 5 mg/mL |
Encapsulation Efficiency | ~30% when loaded in PDEAEMA nanoparticles |
Translocation Rate | Increased with longer alkyl chains; significant across lipid membranes |
Properties
IUPAC Name |
[6-(diethylamino)-9-(2-octadecoxycarbonylphenyl)xanthen-3-ylidene]-diethylazanium;perchlorate | |
---|---|---|
Source | PubChem | |
URL | https://pubchem.ncbi.nlm.nih.gov | |
Description | Data deposited in or computed by PubChem | |
InChI |
InChI=1S/C46H67N2O3.ClHO4/c1-6-11-12-13-14-15-16-17-18-19-20-21-22-23-24-27-34-50-46(49)40-29-26-25-28-39(40)45-41-32-30-37(47(7-2)8-3)35-43(41)51-44-36-38(31-33-42(44)45)48(9-4)10-5;2-1(3,4)5/h25-26,28-33,35-36H,6-24,27,34H2,1-5H3;(H,2,3,4,5)/q+1;/p-1 | |
Source | PubChem | |
URL | https://pubchem.ncbi.nlm.nih.gov | |
Description | Data deposited in or computed by PubChem | |
InChI Key |
WPBUPFBTCDYKNM-UHFFFAOYSA-M | |
Source | PubChem | |
URL | https://pubchem.ncbi.nlm.nih.gov | |
Description | Data deposited in or computed by PubChem | |
Canonical SMILES |
CCCCCCCCCCCCCCCCCCOC(=O)C1=CC=CC=C1C2=C3C=CC(=[N+](CC)CC)C=C3OC4=C2C=CC(=C4)N(CC)CC.[O-]Cl(=O)(=O)=O | |
Source | PubChem | |
URL | https://pubchem.ncbi.nlm.nih.gov | |
Description | Data deposited in or computed by PubChem | |
Molecular Formula |
C46H67ClN2O7 | |
Source | PubChem | |
URL | https://pubchem.ncbi.nlm.nih.gov | |
Description | Data deposited in or computed by PubChem | |
DSSTOX Substance ID |
DTXSID00584976 | |
Record name | 6-(Diethylamino)-N,N-diethyl-9-{2-[(octadecyloxy)carbonyl]phenyl}-3H-xanthen-3-iminium perchlorate | |
Source | EPA DSSTox | |
URL | https://comptox.epa.gov/dashboard/DTXSID00584976 | |
Description | DSSTox provides a high quality public chemistry resource for supporting improved predictive toxicology. | |
Molecular Weight |
795.5 g/mol | |
Source | PubChem | |
URL | https://pubchem.ncbi.nlm.nih.gov | |
Description | Data deposited in or computed by PubChem | |
CAS No. |
142179-00-8 | |
Record name | 6-(Diethylamino)-N,N-diethyl-9-{2-[(octadecyloxy)carbonyl]phenyl}-3H-xanthen-3-iminium perchlorate | |
Source | EPA DSSTox | |
URL | https://comptox.epa.gov/dashboard/DTXSID00584976 | |
Description | DSSTox provides a high quality public chemistry resource for supporting improved predictive toxicology. | |
Record name | Rhodamine B octadecyl ester perchlorate | |
Source | European Chemicals Agency (ECHA) | |
URL | https://echa.europa.eu/information-on-chemicals | |
Description | The European Chemicals Agency (ECHA) is an agency of the European Union which is the driving force among regulatory authorities in implementing the EU's groundbreaking chemicals legislation for the benefit of human health and the environment as well as for innovation and competitiveness. | |
Explanation | Use of the information, documents and data from the ECHA website is subject to the terms and conditions of this Legal Notice, and subject to other binding limitations provided for under applicable law, the information, documents and data made available on the ECHA website may be reproduced, distributed and/or used, totally or in part, for non-commercial purposes provided that ECHA is acknowledged as the source: "Source: European Chemicals Agency, http://echa.europa.eu/". Such acknowledgement must be included in each copy of the material. ECHA permits and encourages organisations and individuals to create links to the ECHA website under the following cumulative conditions: Links can only be made to webpages that provide a link to the Legal Notice page. | |
Retrosynthesis Analysis
AI-Powered Synthesis Planning: Our tool employs the Template_relevance Pistachio, Template_relevance Bkms_metabolic, Template_relevance Pistachio_ringbreaker, Template_relevance Reaxys, Template_relevance Reaxys_biocatalysis model, leveraging a vast database of chemical reactions to predict feasible synthetic routes.
One-Step Synthesis Focus: Specifically designed for one-step synthesis, it provides concise and direct routes for your target compounds, streamlining the synthesis process.
Accurate Predictions: Utilizing the extensive PISTACHIO, BKMS_METABOLIC, PISTACHIO_RINGBREAKER, REAXYS, REAXYS_BIOCATALYSIS database, our tool offers high-accuracy predictions, reflecting the latest in chemical research and data.
Strategy Settings
Precursor scoring | Relevance Heuristic |
---|---|
Min. plausibility | 0.01 |
Model | Template_relevance |
Template Set | Pistachio/Bkms_metabolic/Pistachio_ringbreaker/Reaxys/Reaxys_biocatalysis |
Top-N result to add to graph | 6 |
Feasible Synthetic Routes
Q1: How is Rhodamine B octadecyl ester perchlorate used to study clay minerals?
A1: this compound is utilized as a fluorescent marker in studying the size and shape of clay mineral layers. Researchers create hybrid Langmuir-Blodgett (LB) films by combining the dye with clay minerals like saponite, hectorite, Wyoming bentonite, and Laponite []. The dye helps visualize the clay layers under atomic force microscopy (AFM), revealing their morphology and arrangement within the LB films. This technique provides valuable insights into the structure of these materials, which is crucial for understanding their properties and potential applications in nanocomposites and ultrathin films. [] [1]: https://www.semanticscholar.org/paper/39a33c784bc11e3730e8526b55072b9a35d11f57
Q2: Can this compound be used in sensor applications?
A2: Yes, this compound has shown promise as a component in optical nitrite sensors []. The dye acts as a potential-sensitive dye (PSD) within a plasticized PVC membrane containing a nitrite-selective carrier (benzylbis(triphenylphosphine) palladium(II)chloride). When exposed to nitrite, the fluorescence intensity of the dye increases, providing a measurable signal proportional to nitrite concentration. This sensing mechanism allows for nitrite detection in the 5 to 5000 mg l–1 range. [] [2]: https://www.semanticscholar.org/paper/649a636207eb37d8e17545f8babb47cbb2e6374d
Q3: What are the limitations of using this compound in sensor applications?
A3: While promising for nitrite sensing, this compound presents some limitations. Firstly, the sensor exhibits pH cross-sensitivity, meaning the signal response to nitrite is influenced by the pH of the solution. Secondly, the sensor's lifetime is limited by the gradual leaching of the dye from the membrane, impacting its long-term stability and performance. [] [2]: https://www.semanticscholar.org/paper/649a636207eb37d8e17545f8babb47cbb2e6374d
Disclaimer and Information on In-Vitro Research Products
Please be aware that all articles and product information presented on BenchChem are intended solely for informational purposes. The products available for purchase on BenchChem are specifically designed for in-vitro studies, which are conducted outside of living organisms. In-vitro studies, derived from the Latin term "in glass," involve experiments performed in controlled laboratory settings using cells or tissues. It is important to note that these products are not categorized as medicines or drugs, and they have not received approval from the FDA for the prevention, treatment, or cure of any medical condition, ailment, or disease. We must emphasize that any form of bodily introduction of these products into humans or animals is strictly prohibited by law. It is essential to adhere to these guidelines to ensure compliance with legal and ethical standards in research and experimentation.