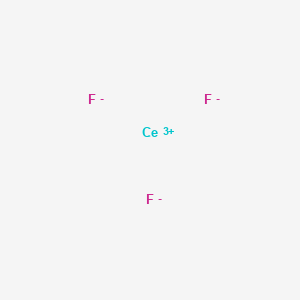
Cerium trifluoride
- Click on QUICK INQUIRY to receive a quote from our team of experts.
- With the quality product at a COMPETITIVE price, you can focus more on your research.
Overview
Description
Cerium trifluoride is an inorganic compound with the chemical formula CeF₄. It is a strong oxidant that appears as a white crystalline material. This compound exists in both anhydrous and monohydrate forms. This compound is known for its high melting point and density, making it a valuable material in various industrial and scientific applications .
Preparation Methods
Cerium trifluoride can be synthesized through several methods:
Fluorination of Cerium(III) Fluoride or Cerium Dioxide: This method involves reacting cerium(III) fluoride or cerium dioxide with fluorine gas at 500°C.
Hydrofluoric Acid Reaction: The hydrated form of cerium fluoride (CeF₄·xH₂O, x≤1) can be produced by reacting 40% hydrofluoric acid with cerium(IV) sulfate solution at 90°C.
Industrial Production: Industrially, cerium fluoride is produced using similar methods but on a larger scale, ensuring high purity and consistency for various applications.
Chemical Reactions Analysis
Cerium trifluoride undergoes several types of chemical reactions:
Oxidation: this compound is a strong oxidizing agent and can oxidize various substrates.
Reduction: It can be reduced to cerium(III) fluoride under specific conditions.
Substitution: This compound can react with other halides to form different cerium halide compounds.
Common reagents used in these reactions include fluorine gas, hydrofluoric acid, and other halides. The major products formed from these reactions are typically other cerium halides or oxides .
Scientific Research Applications
Catalytic Applications
Emission Reduction in Automotive Industry
Cerium trifluoride plays a vital role in catalytic converters, where it helps reduce harmful emissions from vehicle exhaust systems. Its ability to facilitate the conversion of carbon monoxide and nitrogen oxides into less harmful substances is crucial for meeting environmental regulations. Recent studies indicate that this compound enhances the efficiency of catalysts used in these systems, thereby improving fuel economy and reducing emissions .
Catalysis in Organic Reactions
In synthetic organic chemistry, this compound is utilized as a catalyst for various reactions. It promotes reactions involving fluorination and oxidation, making it valuable in the synthesis of pharmaceuticals and agrochemicals. The unique electronic properties of cerium ions enable effective electron transfer processes, enhancing reaction rates and selectivity .
Optical Applications
Scintillation Materials
this compound is recognized for its potential as a scintillator material in high-energy physics experiments. Its high density and fast response times make it suitable for detecting radiation. Research has shown that CeF₃ exhibits excellent radiation resistance, making it a preferred choice for next-generation scintillation detectors .
Up-Conversion Luminescence
The compound demonstrates promising up-conversion luminescence properties, which are harnessed in photonic devices and displays. This compound nanoparticles can be engineered to emit light at different wavelengths when excited by infrared radiation, making them useful in advanced display technologies and biological imaging applications .
Materials Science
Nanostructured Materials
Recent advancements have led to the synthesis of nanoscale this compound polycrystals with tailored morphologies. These nanomaterials exhibit enhanced surface area and reactivity compared to their bulk counterparts. Such properties are beneficial in applications ranging from catalysis to drug delivery systems .
Glass and Coating Applications
this compound is also employed in the production of high-quality optical glasses due to its ability to improve refractive indices and optical clarity. Its application in thin-film coatings enhances the durability and performance of optical devices, including lenses and prisms .
Case Studies
Mechanism of Action
The mechanism by which cerium fluoride exerts its effects involves its strong oxidizing properties. In biological systems, cerium fluoride nanoparticles can prevent the formation of reactive oxygen species, thereby protecting cells from oxidative stress. This property is particularly useful in protecting normal cells during radiation therapy while inducing cell death in cancer cells .
Comparison with Similar Compounds
Cerium trifluoride can be compared with other cerium halides such as cerium chloride (CeCl₃) and cerium bromide (CeBr₃). While all these compounds share similar chemical properties, cerium fluoride is unique due to its higher oxidation state and stronger oxidizing ability. This makes it more effective in certain applications, such as in fluorination reactions and as a catalyst .
Similar compounds include:
- Cerium chloride (CeCl₃)
- Cerium bromide (CeBr₃)
- Cerium iodide (CeI₃)
Each of these compounds has its own unique properties and applications, but cerium fluoride stands out due to its strong oxidizing nature and versatility in various scientific and industrial applications .
Biological Activity
Cerium trifluoride (CeF₃) has garnered attention in recent years for its potential biological activities, particularly in the fields of radioprotection and antioxidant effects. This article synthesizes current research findings, including case studies and data tables, to provide a comprehensive overview of the biological activity of CeF₃.
Overview of this compound
This compound is an inorganic compound that belongs to the group of cerium fluorides. It is primarily known for its applications in materials science but has recently been studied for its biological properties, particularly in the context of nanotechnology.
Biological Mechanisms and Effects
1. Antioxidant Properties
Research indicates that CeF₃ nanoparticles exhibit significant antioxidant activity. They can effectively scavenge reactive oxygen species (ROS), such as hydrogen peroxide, which are often produced during oxidative stress. For example, in a study involving irradiated aqueous solutions, CeF₃ nanoparticles were shown to prevent the formation of hydrogen peroxide and hydroxyl radicals, thus protecting cells from oxidative damage .
2. Radioprotective Effects
CeF₃ has demonstrated a protective effect against radiation-induced damage. In experiments with human mesenchymal stem cells (hMSC), CeF₃ nanoparticles increased cell viability and mitochondrial membrane potential after exposure to radiation. The nanoparticles reduced the number of double-strand breaks (DSBs) in hMSC while inducing cell death in cancer cells (e.g., MCF-7 line) through mitochondrial hyperpolarization . This dual activity suggests that CeF₃ may selectively protect normal cells while targeting cancerous cells.
3. Mitogenic Activity
Interestingly, CeF₃ nanoparticles have been identified as inorganic mitogens, promoting cell proliferation in specific contexts. In studies involving planarians (Schmidtea mediterranea), CeF₃ enhanced blastema regeneration following ionizing irradiation by improving mitotic activity and survival rates of neoblasts, which are crucial for tissue regeneration . This regenerative capacity could have implications for wound healing and tissue engineering.
Case Study 1: Radioprotection in Stem Cells
In a controlled laboratory setting, hMSCs were treated with CeF₃ before being subjected to X-ray irradiation. The results showed:
- Cell Viability : Increased by approximately 40% compared to untreated controls.
- Mitochondrial Potential : Enhanced significantly, indicating improved cellular health.
- DSB Reduction : The number of γ-H2AX foci decreased markedly, demonstrating reduced DNA damage .
Case Study 2: Regeneration in Planarians
Planarians treated with CeF₃ nanoparticles before irradiation exhibited:
- Blastema Growth : Increased by up to 30% compared to controls.
- Mitotic Activity : Retained at 30% post-irradiation, compared to significantly lower levels in untreated groups .
Table 1: Summary of Biological Effects of CeF₃ Nanoparticles
Q & A
Basic Research Questions
Q. What are the key physicochemical properties of cerium trifluoride critical for experimental design?
this compound (CeF₃) exhibits a hexagonal crystal structure (lattice parameters a = 71.1 nm, c = 72.7 nm) with a density of 6.13–6.16 g/cm³ at 25°C . Its high melting point (1703 K) and thermal stability up to ~2600 K make it suitable for high-temperature applications, such as radiation shielding or optical coatings . Key properties include ionic conductivity and fast scintillation response, which are critical for sensor or photonic device development .
Q. What synthesis methods are recommended for high-purity CeF₃ in academic research?
The anhydrous gaseous hydrofluorination method is widely used for synthesizing high-purity CeF₃ (>99.95%). This involves reacting cerium carbonate (Ce₂(CO₃)₃) or cerium oxide (CeO₂) with hydrogen fluoride (HF) in a continuous-mode screw reactor at 200–550°C . Reaction kinetics depend on raw material properties (e.g., specific surface area and dispersion of CeCO₃) and process variables (e.g., HF flow rate and residence time) . For nanoscale polycrystals, ammonium hydrogen difluoride (NH₄HF₂) can serve as a fluorinating agent under controlled thermal conditions .
Q. How can researchers characterize CeF₃ crystal structure and purity?
- X-ray diffraction (XRD): Confirms hexagonal phase purity and lattice parameters .
- Infrared (IR) spectroscopy: Detects moisture content (0.04–0.07 mass%) and hydroxyl impurities .
- Thermogravimetric analysis (TGA): Assesses thermal stability and decomposition behavior .
- Inductively coupled plasma mass spectrometry (ICP-MS): Quantifies trace impurities (e.g., divalent cations like Ca²⁺ or Mg²⁺, which introduce defects) .
Advanced Research Questions
Q. How do trace impurities in CeF₃ affect its scintillation performance, and how can these be mitigated?
Divalent cations (e.g., Ca²⁺) disrupt charge compensation in the CeF₃ lattice, increasing defect density and reducing scintillation efficiency . Advanced purification techniques, such as zone refining or vacuum sublimation, are required to achieve >99.999% purity. Doping with Tb³⁺, Pr³⁺, or Yb³⁺ enhances luminescence by modifying the energy transfer pathways . Researchers must balance dopant concentration (typically 0.1–1.0 mol%) to avoid quenching effects .
Q. What experimental strategies resolve contradictions in reported thermal expansion coefficients of CeF₃?
Discrepancies in thermal expansion data often arise from differences in sample purity, measurement techniques (e.g., dilatometry vs. XRD), or anisotropic crystal behavior. To address this:
- Use single-crystal samples to isolate directional thermal expansion (hexagonal a- and c-axes) .
- Validate results with in situ high-temperature XRD to track lattice parameter changes .
- Cross-reference data with NIST standards or computational models (e.g., density functional theory) .
Q. How can computational modeling optimize CeF₃ for energy storage or sensor applications?
First-principles calculations (e.g., DFT) predict electronic band structures, defect formation energies, and ion migration pathways. For example:
- Ce³⁺ vacancy migration barriers determine ionic conductivity in solid-state electrolytes .
- Simulated dopant interactions with CeF₃ lattices guide experimental designs for enhanced optical response . Pair computational results with experimental validation using impedance spectroscopy or photoluminescence mapping .
Q. What are the challenges in scaling CeF₃ synthesis from lab to pilot-scale production?
Key challenges include:
- Reactor design: Horizontal screw reactors must maintain uniform temperature and HF distribution to prevent incomplete fluorination .
- Safety protocols: HF handling requires corrosion-resistant materials (e.g., nickel alloys) and real-time gas monitoring .
- Cost optimization: Raw material selection (CeO₂ vs. CeCO₃) impacts process efficiency and final product cost (estimated at ~30 USD/kg for 99.95% purity) .
Q. Methodological Guidance for Researchers
Q. How to design experiments analyzing CeF₃’s stability under extreme conditions?
- High-temperature studies: Use thermocouple-controlled furnaces with inert gas (Ar) to prevent oxidation. Monitor phase transitions via differential scanning calorimetry (DSC) .
- Radiation exposure tests: Irradiate CeF₃ samples with γ-rays or protons, then evaluate structural damage using Raman spectroscopy or positron annihilation lifetime spectroscopy (PALS) .
Q. What statistical approaches are recommended for validating CeF₃ property data?
Apply multivariate regression to correlate synthesis parameters (e.g., HF concentration, reaction time) with material properties (e.g., purity, particle size). Use ANOVA to identify significant variables and reduce experimental noise . For reproducibility, report confidence intervals (95% CI) and measurement uncertainties (e.g., ±0.02 g/cm³ for density) .
Properties
CAS No. |
7758-88-5 |
---|---|
Molecular Formula |
CeF3 |
Molecular Weight |
197.111 g/mol |
IUPAC Name |
trifluorocerium |
InChI |
InChI=1S/Ce.3FH/h;3*1H/q+3;;;/p-3 |
InChI Key |
QCCDYNYSHILRDG-UHFFFAOYSA-K |
SMILES |
[F-].[F-].[F-].[Ce+3] |
Canonical SMILES |
F[Ce](F)F |
Key on ui other cas no. |
37317-01-4 7758-88-5 |
physical_description |
Hexagonal crystals or powder; [Merck Index] Insoluble in water and acids; [Hawley] |
Pictograms |
Irritant |
Synonyms |
cerium fluoride cerium trifluoride cerous fluoride |
Origin of Product |
United States |
Disclaimer and Information on In-Vitro Research Products
Please be aware that all articles and product information presented on BenchChem are intended solely for informational purposes. The products available for purchase on BenchChem are specifically designed for in-vitro studies, which are conducted outside of living organisms. In-vitro studies, derived from the Latin term "in glass," involve experiments performed in controlled laboratory settings using cells or tissues. It is important to note that these products are not categorized as medicines or drugs, and they have not received approval from the FDA for the prevention, treatment, or cure of any medical condition, ailment, or disease. We must emphasize that any form of bodily introduction of these products into humans or animals is strictly prohibited by law. It is essential to adhere to these guidelines to ensure compliance with legal and ethical standards in research and experimentation.