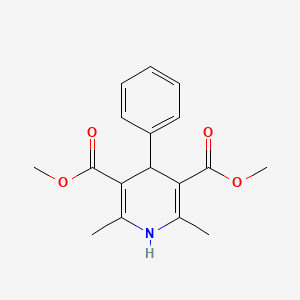
Dimethyl 2,6-dimethyl-4-phenyl-1,4-dihydropyridine-3,5-dicarboxylate
Overview
Description
Dimethyl 2,6-dimethyl-4-phenyl-1,4-dihydropyridine-3,5-dicarboxylate is a compound belonging to the class of 1,4-dihydropyridines. These compounds are known for their diverse biological activities and are often used as building blocks in organic synthesis for the preparation of various biologically active compounds .
Preparation Methods
Synthetic Routes and Reaction Conditions
The synthesis of Dimethyl 2,6-dimethyl-4-phenyl-1,4-dihydropyridine-3,5-dicarboxylate typically involves the Hantzsch reaction. This multicomponent reaction involves the condensation of an aldehyde, a β-keto ester, and ammonia or an ammonium salt. The reaction conditions often include the use of ethanol as a solvent and heating under reflux .
Industrial Production Methods
In industrial settings, the synthesis of this compound can be scaled up using similar reaction conditions but with optimized parameters to ensure high yield and purity. The use of continuous flow reactors and other advanced technologies can further enhance the efficiency of the production process .
Chemical Reactions Analysis
Types of Reactions
Dimethyl 2,6-dimethyl-4-phenyl-1,4-dihydropyridine-3,5-dicarboxylate undergoes various chemical reactions, including:
Oxidation: This compound can be oxidized to form pyridine derivatives.
Reduction: It can be reduced to form tetrahydropyridine derivatives.
Substitution: Various substituents can be introduced at different positions on the pyridine ring.
Common Reagents and Conditions
Oxidation: Common oxidizing agents include potassium permanganate and chromium trioxide.
Reduction: Reducing agents such as sodium borohydride and lithium aluminum hydride are often used.
Substitution: Electrophilic and nucleophilic reagents can be used under appropriate conditions to introduce various substituents.
Major Products
The major products formed from these reactions include pyridine derivatives, tetrahydropyridine derivatives, and various substituted dihydropyridines .
Scientific Research Applications
Dimethyl 2,6-dimethyl-4-phenyl-1,4-dihydropyridine-3,5-dicarboxylate has several scientific research applications:
Chemistry: Used as a building block in the synthesis of various biologically active compounds.
Biology: Studied for its potential biological activities, including anti-inflammatory and analgesic properties.
Medicine: Investigated for its potential use in the treatment of cardiovascular diseases due to its calcium channel blocking activity.
Industry: Used in the development of new materials and as a catalyst in various chemical reactions
Mechanism of Action
The mechanism of action of Dimethyl 2,6-dimethyl-4-phenyl-1,4-dihydropyridine-3,5-dicarboxylate involves its interaction with calcium channels. By blocking these channels, it can modulate calcium influx into cells, which is crucial for various physiological processes. This mechanism is particularly relevant in its potential use as a cardiovascular drug .
Comparison with Similar Compounds
Similar Compounds
Nifedipine: A well-known calcium channel blocker used in the treatment of hypertension.
Amlodipine: Another calcium channel blocker with similar applications.
Felodipine: Used for its antihypertensive and antianginal properties
Uniqueness
Dimethyl 2,6-dimethyl-4-phenyl-1,4-dihydropyridine-3,5-dicarboxylate is unique due to its specific structural features, which confer distinct biological activities. Its ability to undergo various chemical reactions also makes it a versatile compound in synthetic chemistry .
Properties
IUPAC Name |
dimethyl 2,6-dimethyl-4-phenyl-1,4-dihydropyridine-3,5-dicarboxylate | |
---|---|---|
Source | PubChem | |
URL | https://pubchem.ncbi.nlm.nih.gov | |
Description | Data deposited in or computed by PubChem | |
InChI |
InChI=1S/C17H19NO4/c1-10-13(16(19)21-3)15(12-8-6-5-7-9-12)14(11(2)18-10)17(20)22-4/h5-9,15,18H,1-4H3 | |
Source | PubChem | |
URL | https://pubchem.ncbi.nlm.nih.gov | |
Description | Data deposited in or computed by PubChem | |
InChI Key |
QDHIAGVDKRWZNF-UHFFFAOYSA-N | |
Source | PubChem | |
URL | https://pubchem.ncbi.nlm.nih.gov | |
Description | Data deposited in or computed by PubChem | |
Canonical SMILES |
CC1=C(C(C(=C(N1)C)C(=O)OC)C2=CC=CC=C2)C(=O)OC | |
Source | PubChem | |
URL | https://pubchem.ncbi.nlm.nih.gov | |
Description | Data deposited in or computed by PubChem | |
Molecular Formula |
C17H19NO4 | |
Source | PubChem | |
URL | https://pubchem.ncbi.nlm.nih.gov | |
Description | Data deposited in or computed by PubChem | |
DSSTOX Substance ID |
DTXSID10908240 | |
Record name | Dimethyl 2,6-dimethyl-4-phenyl-1,4-dihydropyridine-3,5-dicarboxylate | |
Source | EPA DSSTox | |
URL | https://comptox.epa.gov/dashboard/DTXSID10908240 | |
Description | DSSTox provides a high quality public chemistry resource for supporting improved predictive toxicology. | |
Molecular Weight |
301.34 g/mol | |
Source | PubChem | |
URL | https://pubchem.ncbi.nlm.nih.gov | |
Description | Data deposited in or computed by PubChem | |
CAS No. |
70677-78-0, 103183-96-6 | |
Record name | 1,4-Dihydro-2,6-dimethyl-4-phenyl-3,5-pyridinecarboxylic acid dimethyl ester | |
Source | ChemIDplus | |
URL | https://pubchem.ncbi.nlm.nih.gov/substance/?source=chemidplus&sourceid=0070677780 | |
Description | ChemIDplus is a free, web search system that provides access to the structure and nomenclature authority files used for the identification of chemical substances cited in National Library of Medicine (NLM) databases, including the TOXNET system. | |
Record name | Dimethyl 2,6-dimethyl-4-phenyl-1,4-dihydropyridine-3,5-dicarboxylate | |
Source | EPA DSSTox | |
URL | https://comptox.epa.gov/dashboard/DTXSID10908240 | |
Description | DSSTox provides a high quality public chemistry resource for supporting improved predictive toxicology. | |
Record name | 2,6-Dimethyl-4-phenyl-1,4-dihydro-pyridine-3, 5-dicarboxylic acid dimethyl ester | |
Source | European Chemicals Agency (ECHA) | |
URL | https://echa.europa.eu/information-on-chemicals | |
Description | The European Chemicals Agency (ECHA) is an agency of the European Union which is the driving force among regulatory authorities in implementing the EU's groundbreaking chemicals legislation for the benefit of human health and the environment as well as for innovation and competitiveness. | |
Explanation | Use of the information, documents and data from the ECHA website is subject to the terms and conditions of this Legal Notice, and subject to other binding limitations provided for under applicable law, the information, documents and data made available on the ECHA website may be reproduced, distributed and/or used, totally or in part, for non-commercial purposes provided that ECHA is acknowledged as the source: "Source: European Chemicals Agency, http://echa.europa.eu/". Such acknowledgement must be included in each copy of the material. ECHA permits and encourages organisations and individuals to create links to the ECHA website under the following cumulative conditions: Links can only be made to webpages that provide a link to the Legal Notice page. | |
Disclaimer and Information on In-Vitro Research Products
Please be aware that all articles and product information presented on BenchChem are intended solely for informational purposes. The products available for purchase on BenchChem are specifically designed for in-vitro studies, which are conducted outside of living organisms. In-vitro studies, derived from the Latin term "in glass," involve experiments performed in controlled laboratory settings using cells or tissues. It is important to note that these products are not categorized as medicines or drugs, and they have not received approval from the FDA for the prevention, treatment, or cure of any medical condition, ailment, or disease. We must emphasize that any form of bodily introduction of these products into humans or animals is strictly prohibited by law. It is essential to adhere to these guidelines to ensure compliance with legal and ethical standards in research and experimentation.