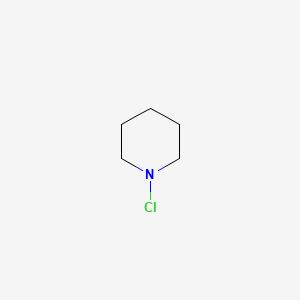
N-Chloropiperidine
Overview
Description
N-Chloropiperidine (CAS 2156-71-0) is an organic chloramine derived from piperidine, a six-membered heterocyclic amine. It is synthesized via the reaction of piperidine with sodium hypochlorite under controlled conditions, typically at low temperatures (0–5°C) to minimize decomposition . The compound is highly volatile and reactive, necessitating immediate use or stabilization in solvents like toluene post-synthesis . Its stability in aqueous solutions is pH-dependent, with a half-life of ~80 hours at pH 7.0 (25°C) under dark conditions, though photodecomposition accelerates degradation .
It serves as a model compound for understanding the behavior of organic chloramines in both synthetic and environmental contexts.
Preparation Methods
Synthetic Routes and Reaction Conditions
N-Chloropiperidine is commonly prepared by treating piperidine with calcium hypochlorite. The reaction is carried out in an aqueous solution at low temperatures to control the reactivity of the chloramine . Another method involves the reaction of piperidine with N-chlorosuccinimide in dichloromethane .
Industrial Production Methods
Industrial production of this compound follows similar synthetic routes but on a larger scale. The process involves careful control of reaction conditions to ensure safety and efficiency. The use of continuous flow reactors can help manage the exothermic nature of the reaction and improve yield .
Chemical Reactions Analysis
Types of Reactions
N-Chloropiperidine undergoes several types of chemical reactions, including:
Dehydrohalogenation: This reaction leads to the formation of cyclic imines.
Substitution Reactions: The nitrogen-chlorine bond can be substituted by various nucleophiles.
Oxidation and Reduction: These reactions can modify the functional groups attached to the piperidine ring.
Common Reagents and Conditions
Dehydrohalogenation: Typically requires a base such as potassium hydroxide in an ethanol solution.
Substitution Reactions: Common reagents include nucleophiles like amines or thiols.
Oxidation and Reduction: Reagents such as hydrogen peroxide for oxidation and sodium borohydride for reduction are commonly used.
Major Products Formed
Cyclic Imines: Formed through dehydrohalogenation.
Substituted Piperidines: Result from substitution reactions.
Scientific Research Applications
Chemical Applications
1. Synthesis of Organic Compounds
N-Chloropiperidine serves as an important intermediate in the synthesis of various organic compounds. Its high reactivity, particularly due to the nitrogen-chlorine bond, makes it valuable in producing agrochemicals and pharmaceuticals. The compound can participate in nucleophilic substitution reactions, contributing to the formation of more complex molecular structures.
2. Base-Catalyzed Reactions
Research indicates that this compound undergoes base-catalyzed rearrangements, which are essential for understanding its reactivity in different environments. Quantum-chemical studies have calculated reaction energies for these processes, providing insights into its stability and potential reaction pathways in both gas and aqueous phases .
Biological Applications
1. Mutagenicity Studies
this compound has been studied for its mutagenic properties. It is known to induce chromosomal aberrations in mammalian cells, making it a subject of interest for genetic research. The compound acts as a direct-acting mutagen, causing mutations through base pair substitutions.
2. Anticancer Potential
Recent investigations into chloropiperidines have revealed their potential as anticancer agents. Specifically, bis-3-chloropiperidines have shown efficacy in alkylating DNA and inducing strand cleavage, presenting a promising avenue for cancer treatment development. These compounds exhibited low nanomolar cytotoxicity against various carcinoma cell lines, outperforming traditional chemotherapy agents like chlorambucil .
Environmental Applications
1. Photocatalytic Degradation
this compound has been subjected to studies focusing on its degradation using photocatalytic techniques. Research demonstrated that titanium dioxide (TiO₂) could effectively degrade this compound under ultraviolet illumination, with degradation rates following first-order kinetics. This finding highlights the compound's relevance in water treatment processes aimed at removing hazardous contaminants .
2. Disinfection By-Products
As a model compound for studying disinfection by-products (DBPs), this compound's stability and reactivity under various pH conditions have been analyzed to assess its implications for human health and environmental safety. Understanding the formation and distribution of such compounds is crucial for managing risks associated with drinking water quality .
Case Studies
Mechanism of Action
N-Chloropiperidine exerts its effects primarily through the formation of highly reactive intermediates, such as aziridinium ions. These intermediates can alkylate nucleophiles, including DNA bases, leading to covalent adduct formation. This mechanism is similar to that of other alkylating agents used in chemotherapy .
Comparison with Similar Compounds
Reactivity and Selectivity in Chlorination Reactions
N-Chloropiperidine exhibits distinct reactivity patterns compared to other N-chloroamines. In trifluoroacetic acid, its chlorination selectivity for para-substituted aromatic substrates (e.g., methoxybenzene) reaches 99%, significantly higher than N-chloromorpholine (20%) or N-chlorodimethylamine (6.0%) (Table 1) . However, its reactivity is comparatively low: N-chloromorpholine is 17,000× more reactive than this compound in chlorinating 2-chloromethoxybenzene . This inverse relationship between reactivity and selectivity highlights its utility in controlled synthetic applications.
Table 1: Reactivity and Selectivity of N-Chloroamines in Trifluoroacetic Acid
N-Chloroamine | Relative Reactivity (vs. NCP) | 4-/2-Chlorination Ratio |
---|---|---|
This compound (NCP) | 1 | 99 |
N-Chloromorpholine | 17,000 | 20 |
N-Chlorodimethylamine | 160,000 | 6.0 |
Stability and Decomposition Pathways
This compound decomposes via two primary pathways:
Spontaneous hydrolysis : Follows first-order kinetics in aqueous solutions, producing piperidine and chloride ions .
Photocatalytic degradation : Ni/NiO-C3N4 catalysts under visible light degrade NCP with >90% efficiency within 2 hours, outperforming TiO2-based systems .
In contrast, N-chlorodiethylamine decomposes faster (t₁/₂ ~40 hours at pH 7.0) due to steric effects enhancing hydrolysis .
Toxicological Profiles
This compound demonstrates direct mutagenicity in Salmonella typhimurium TA100 (2.4× higher revertants than piperidine) and induces chromosomal aberrations in CHO cells, including multipolar anaphase and micronuclei . Chronic exposure in mice causes dominant lethal mutations and sperm abnormalities at doses >50 mg/kg .
Comparatively, N-chlorodimethylamine shows weaker mutagenic activity (1.5× baseline revertants) but higher acute toxicity in rodent models .
Computational and Mechanistic Insights
Quantum-chemical studies reveal that this compound undergoes base-catalyzed dehydrochlorination with a calculated activation energy of 17.0 kcal/mol in aqueous media, matching experimental data . Solvent effects critically influence reaction pathways: explicit water molecules lower the energy barrier for ring inversion by 3–5 kcal/mol compared to gas-phase calculations .
In contrast, N-chloro-3-(hydroxymethyl)piperidine favors intramolecular addition over elimination due to hydrogen-bond stabilization, yielding oxazinane derivatives instead of imines .
Biological Activity
N-Chloropiperidine, a chlorinated derivative of piperidine, has garnered attention for its biological activity, particularly in the context of mutagenicity, cytotoxicity, and interactions with nucleic acids. This article synthesizes findings from various studies to provide a comprehensive overview of its biochemical properties, mechanisms of action, and potential applications.
This compound (C₅H₁₀ClN) is known for its instability, undergoing spontaneous decomposition and photodecomposition in aqueous solutions. This instability can affect its biological activity and the interpretation of experimental results . The compound is characterized by its ability to participate in various biochemical reactions, particularly those involving nucleophilic substitution and alkylation processes.
Enzyme Interactions
This compound interacts with several enzymes and proteins, influencing numerous cellular processes. Its interaction with arenesulfinic acid salts leads to the formation of stable arenesulfonamides, which are important in synthetic organic chemistry. Additionally, it has been shown to induce chromosomal aberrations in cultured mammalian cells, indicating its potential as a mutagen.
DNA Damage and Cytotoxicity
One of the most critical aspects of this compound's biological activity is its effect on DNA. Studies have demonstrated that it acts as a direct-acting mutagen, primarily through base pair substitutions that lead to mutations .
In a comparative study involving chloropiperidines as potential anticancer agents, this compound exhibited varying degrees of cytotoxicity depending on structural modifications. For instance, methyl substituents in 3-chloropiperidines enhanced their reactivity and cytotoxicity towards cancer cell lines .
Table 1 summarizes the cytotoxic effects observed in various studies:
Compound | IC₅₀ (µM) | Cell Line | Observations |
---|---|---|---|
This compound | 75.3 | BxPC-3 | Moderate cytotoxicity compared to chlorambucil |
3-Chloropiperidine-1 | 50 | Pancreatic | Higher cytotoxicity due to structural modifications |
Chlorambucil | 75.3 | BxPC-3 | Reference compound for comparison |
The mutagenic activity of this compound can be attributed to its ability to alkylate DNA. This process involves the formation of DNA adducts that can lead to strand breaks and other forms of damage. For example, studies using plasmid DNA have shown that this compound can induce significant changes in supercoiled plasmid mobility due to alkylation events .
Molecular Mechanisms
The molecular mechanisms underlying the biological effects of this compound involve multiple pathways:
- Direct Alkylation : The compound can directly interact with nucleobases in DNA, leading to modifications that disrupt normal replication processes.
- Enzyme-Mediated Damage : It has been shown to induce damage through topoisomerase II-mediated pathways, which are critical for maintaining DNA topology during replication .
Case Studies
Several case studies have highlighted the implications of this compound's biological activity:
- Cytotoxicity Assessment : In one study, various chlorinated piperidines were tested for their cytotoxic effects on pancreatic cancer cell lines. The results indicated that structural variations significantly influenced their potency, with some derivatives exhibiting low nanomolar IC₅₀ values .
- Environmental Impact : Research has also focused on the environmental degradation pathways of this compound when released into water systems. Its chlorinated derivatives can undergo transformations that affect aquatic ecosystems and human health due to their mutagenic properties .
Q & A
Basic Research Questions
Q. What experimental parameters optimize the synthesis of N-chloropiperidine in flow reactors?
- Methodological Answer : High-yield synthesis (e.g., >90%) can be achieved using well-mixed meso-scale flow reactors with static mixers. Key parameters include short residence times (e.g., <5 minutes), controlled molar equivalents of NaOCl (1.05–1.1 equiv), and reactor volumes adjusted to amine reactivity. For example, N-chloropiperidine synthesis benefits from reduced side reactions under continuous flow conditions compared to batch processes .
Q. How can N-chloropiperidine stability in aqueous solutions be systematically assessed?
- Methodological Answer : Stability studies should account for both spontaneous decomposition (pH-dependent hydrolysis) and photodecomposition. Use buffered solutions (e.g., phosphate buffer at pH 7–9) and monitor degradation via UV-Vis spectroscopy or HPLC. For photostability, expose samples to UV light (254 nm) and quantify residual N-chloropiperidine using derivatization with aryl sulfonic acid salts, which form stable sulfonamides for analysis .
Q. What analytical methods are recommended for detecting trace N-chloropiperidine in complex matrices?
- Methodological Answer : Derivatization with dansyl chloride (DANSO, H) followed by reverse-phase HPLC with fluorescence detection provides high sensitivity (detection limit ~10 ng). Adjust pH to 9.5–10 during derivatization to maximize yield, and employ solid-phase extraction (e.g., SEP-PAK cartridges) to concentrate samples. Calibration curves should span 10⁻⁷–10⁻⁶ M for environmental or biological matrices .
Advanced Research Questions
Q. How do base-catalyzed degradation pathways of N-chloropiperidine differ in chlorinated water systems?
- Methodological Answer : Computational quantum-chemical studies (e.g., DFT calculations) reveal that hydroxide ions promote nucleophilic substitution at the N–Cl bond, forming piperidine and chloride. Competing pathways, such as Hofmann elimination or rearrangement, depend on solvent polarity and temperature. Validate predictions experimentally via GC-MS analysis of degradation products under controlled pH (e.g., pH 10–12) .
Q. What strategies resolve contradictions in reported yields of N-chloropiperidine-derived products in radical addition reactions?
- Methodological Answer : Discrepancies (e.g., low yields in chromous chloride-mediated reactions) may arise from competing termination steps (e.g., Cr²⁺ reducing intermediate radicals). Use radical traps (e.g., TEMPO) to confirm mechanistic pathways. Optimize reaction conditions by varying initiator concentrations and monitoring intermediates via EPR spectroscopy .
Q. How can N-chloropiperidine’s competitive chlorination kinetics be modeled in biological matrices like stomach fluid?
- Methodological Answer : Simulate gastric environments using in vitro models with physiologically relevant amines (e.g., glycine, histidine) and hypochlorite. Quantify N-chloropiperidine formation via LC-MS/MS and apply kinetic models (e.g., second-order rate constants) to assess competition. Account for pH effects (stomach pH ~1.5–3.5) and competing reactions with biomolecules .
Q. What toxicological models are appropriate for assessing N-chloropiperidine’s bioaccumulation potential?
- Methodological Answer : Use in vivo rodent studies with sequential dosing of piperidine and NaOCl to mimic endogenous formation. Measure blood and tissue concentrations via isotopic labeling (e.g., ¹⁴C-piperidine) and assess metabolites via high-resolution mass spectrometry. Compare results to structurally similar N-chloroamines (e.g., N-chloromorpholine) to identify structure-toxicity relationships .
Q. Data Contradiction & Replication Guidance
- Key Consideration : Replicate studies using protocols from primary literature (e.g., derivatization pH, reactor configurations) and validate via interlaboratory comparisons. For conflicting stability data, re-examine environmental variables (light exposure, trace metal contaminants) .
- Statistical Rigor : Report confidence intervals for kinetic constants and use ANOVA to compare degradation rates across experimental conditions .
Properties
IUPAC Name |
1-chloropiperidine | |
---|---|---|
Source | PubChem | |
URL | https://pubchem.ncbi.nlm.nih.gov | |
Description | Data deposited in or computed by PubChem | |
InChI |
InChI=1S/C5H10ClN/c6-7-4-2-1-3-5-7/h1-5H2 | |
Source | PubChem | |
URL | https://pubchem.ncbi.nlm.nih.gov | |
Description | Data deposited in or computed by PubChem | |
InChI Key |
CIQJWKNJDQKPPO-UHFFFAOYSA-N | |
Source | PubChem | |
URL | https://pubchem.ncbi.nlm.nih.gov | |
Description | Data deposited in or computed by PubChem | |
Canonical SMILES |
C1CCN(CC1)Cl | |
Source | PubChem | |
URL | https://pubchem.ncbi.nlm.nih.gov | |
Description | Data deposited in or computed by PubChem | |
Molecular Formula |
C5H10ClN | |
Source | PubChem | |
URL | https://pubchem.ncbi.nlm.nih.gov | |
Description | Data deposited in or computed by PubChem | |
DSSTOX Substance ID |
DTXSID10175894 | |
Record name | N-Chloropiperidine | |
Source | EPA DSSTox | |
URL | https://comptox.epa.gov/dashboard/DTXSID10175894 | |
Description | DSSTox provides a high quality public chemistry resource for supporting improved predictive toxicology. | |
Molecular Weight |
119.59 g/mol | |
Source | PubChem | |
URL | https://pubchem.ncbi.nlm.nih.gov | |
Description | Data deposited in or computed by PubChem | |
CAS No. |
2156-71-0 | |
Record name | 1-Chloropiperidine | |
Source | CAS Common Chemistry | |
URL | https://commonchemistry.cas.org/detail?cas_rn=2156-71-0 | |
Description | CAS Common Chemistry is an open community resource for accessing chemical information. Nearly 500,000 chemical substances from CAS REGISTRY cover areas of community interest, including common and frequently regulated chemicals, and those relevant to high school and undergraduate chemistry classes. This chemical information, curated by our expert scientists, is provided in alignment with our mission as a division of the American Chemical Society. | |
Explanation | The data from CAS Common Chemistry is provided under a CC-BY-NC 4.0 license, unless otherwise stated. | |
Record name | N-Chloropiperidine | |
Source | ChemIDplus | |
URL | https://pubchem.ncbi.nlm.nih.gov/substance/?source=chemidplus&sourceid=0002156710 | |
Description | ChemIDplus is a free, web search system that provides access to the structure and nomenclature authority files used for the identification of chemical substances cited in National Library of Medicine (NLM) databases, including the TOXNET system. | |
Record name | N-Chloropiperidine | |
Source | EPA DSSTox | |
URL | https://comptox.epa.gov/dashboard/DTXSID10175894 | |
Description | DSSTox provides a high quality public chemistry resource for supporting improved predictive toxicology. | |
Record name | 1-CHLOROPIPERIDINE | |
Source | FDA Global Substance Registration System (GSRS) | |
URL | https://gsrs.ncats.nih.gov/ginas/app/beta/substances/4V5HK98PCN | |
Description | The FDA Global Substance Registration System (GSRS) enables the efficient and accurate exchange of information on what substances are in regulated products. Instead of relying on names, which vary across regulatory domains, countries, and regions, the GSRS knowledge base makes it possible for substances to be defined by standardized, scientific descriptions. | |
Explanation | Unless otherwise noted, the contents of the FDA website (www.fda.gov), both text and graphics, are not copyrighted. They are in the public domain and may be republished, reprinted and otherwise used freely by anyone without the need to obtain permission from FDA. Credit to the U.S. Food and Drug Administration as the source is appreciated but not required. | |
Retrosynthesis Analysis
AI-Powered Synthesis Planning: Our tool employs the Template_relevance Pistachio, Template_relevance Bkms_metabolic, Template_relevance Pistachio_ringbreaker, Template_relevance Reaxys, Template_relevance Reaxys_biocatalysis model, leveraging a vast database of chemical reactions to predict feasible synthetic routes.
One-Step Synthesis Focus: Specifically designed for one-step synthesis, it provides concise and direct routes for your target compounds, streamlining the synthesis process.
Accurate Predictions: Utilizing the extensive PISTACHIO, BKMS_METABOLIC, PISTACHIO_RINGBREAKER, REAXYS, REAXYS_BIOCATALYSIS database, our tool offers high-accuracy predictions, reflecting the latest in chemical research and data.
Strategy Settings
Precursor scoring | Relevance Heuristic |
---|---|
Min. plausibility | 0.01 |
Model | Template_relevance |
Template Set | Pistachio/Bkms_metabolic/Pistachio_ringbreaker/Reaxys/Reaxys_biocatalysis |
Top-N result to add to graph | 6 |
Feasible Synthetic Routes
Disclaimer and Information on In-Vitro Research Products
Please be aware that all articles and product information presented on BenchChem are intended solely for informational purposes. The products available for purchase on BenchChem are specifically designed for in-vitro studies, which are conducted outside of living organisms. In-vitro studies, derived from the Latin term "in glass," involve experiments performed in controlled laboratory settings using cells or tissues. It is important to note that these products are not categorized as medicines or drugs, and they have not received approval from the FDA for the prevention, treatment, or cure of any medical condition, ailment, or disease. We must emphasize that any form of bodily introduction of these products into humans or animals is strictly prohibited by law. It is essential to adhere to these guidelines to ensure compliance with legal and ethical standards in research and experimentation.