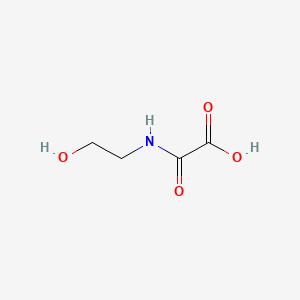
Hydroxyethyloxamic acid
Overview
Description
Hydroxyethyloxamic acid, also known as N-(2-hydroxyethyl)oxamic acid, is an organic compound with the molecular formula C4H7NO4. It is a derivative of oxamic acid, where one of the hydrogen atoms is replaced by a hydroxyethyl group.
Mechanism of Action
Target of Action
Hydroxyethyloxamic acid is a metabolite of metronidazole, a commonly used antibiotic . Metronidazole targets anaerobic or microaerophilic microorganisms . The redox potential of the electron transport portions of these organisms renders metronidazole selective to these organisms, which cause nitro group reduction, leading to the production of toxic metabolites .
Mode of Action
It is believed that an intermediate in the reduction of metronidazole, which is only made by anaerobic bacteria and protozoa, binds deoxyribonucleic acid and electron-transport proteins of organisms, blocking nucleic acid synthesis . These include this compound and acetamide, which may damage DNA of replicating organisms .
Biochemical Pathways
It is known that metronidazole and its metabolites, including this compound, can interfere with the dna synthesis of anaerobic bacteria and protozoa . This interference can lead to cell death and the elimination of the infection .
Pharmacokinetics
Metronidazole, from which this compound is derived, is known to be well-absorbed after oral administration and is distributed widely in body tissues . It is metabolized in the liver and excreted in the urine
Result of Action
The primary result of the action of this compound is the disruption of DNA synthesis in anaerobic bacteria and protozoa, leading to cell death . This can result in the elimination of the infection.
Action Environment
The action environment of this compound is likely to be within the cells of anaerobic bacteria and protozoa, where it interferes with DNA synthesis
Biochemical Analysis
Biochemical Properties
Hydroxyethyloxamic acid plays a significant role in biochemical reactions due to its ability to form stable complexes with metal ions . It interacts with several enzymes, proteins, and other biomolecules through chelation and hydrogen bonding . Notably, this compound can inhibit enzymes such as matrix metalloproteinases, angiotensin-converting enzyme, and histone deacetylase . These interactions are crucial for its potential therapeutic applications, including cancer treatment and cardiovascular disease management .
Cellular Effects
This compound influences various cellular processes, including cell signaling pathways, gene expression, and cellular metabolism . It has been observed to affect cell stress and cytotoxicity responses, which can lead to changes in cell viability and function . Additionally, this compound can modulate the activity of specific biomolecular targets, thereby impacting cellular homeostasis and metabolic pathways .
Molecular Mechanism
At the molecular level, this compound exerts its effects through binding interactions with biomolecules and enzyme inhibition . It can chelate metal ions, which is essential for its inhibitory action on enzymes such as matrix metalloproteinases and histone deacetylase . These interactions lead to changes in gene expression and cellular function, contributing to its therapeutic potential .
Temporal Effects in Laboratory Settings
In laboratory settings, the effects of this compound can vary over time due to its stability and degradation . Studies have shown that the compound remains stable under specific conditions, but prolonged exposure to certain temperatures and pH levels can lead to its degradation . Long-term effects on cellular function have been observed in both in vitro and in vivo studies, highlighting the importance of monitoring its stability during experiments .
Dosage Effects in Animal Models
The effects of this compound in animal models are dose-dependent . At lower dosages, the compound exhibits therapeutic benefits, such as enzyme inhibition and modulation of cellular processes . At higher dosages, toxic or adverse effects may occur, including cytotoxicity and disruption of cellular homeostasis . These findings underscore the need for careful dosage optimization in preclinical studies .
Metabolic Pathways
This compound is involved in various metabolic pathways, including those related to its chelating properties and enzyme interactions . It interacts with enzymes such as carbonic anhydrase and urease, affecting metabolic flux and metabolite levels . These interactions are critical for understanding its role in cellular metabolism and potential therapeutic applications .
Transport and Distribution
Within cells and tissues, this compound is transported and distributed through passive and active transport mechanisms . It interacts with transporters and binding proteins, influencing its localization and accumulation in specific cellular compartments . These interactions are essential for its bioavailability and efficacy in therapeutic applications .
Subcellular Localization
This compound exhibits specific subcellular localization, which can affect its activity and function . It is directed to particular compartments or organelles through targeting signals and post-translational modifications . Understanding its subcellular localization is crucial for elucidating its mechanism of action and optimizing its therapeutic potential .
Preparation Methods
Synthetic Routes and Reaction Conditions: Hydroxyethyloxamic acid can be synthesized through several methods. One common approach involves the reaction of ethyl oxalate with ethanolamine under controlled conditions. The reaction typically proceeds as follows: [ \text{C2H5OCOCOOC2H5} + \text{HOCH2CH2NH2} \rightarrow \text{HOCH2CH2NHCOCOOH} + \text{C2H5OH} ]
Industrial Production Methods: In industrial settings, this compound can be produced using similar synthetic routes but on a larger scale. The reaction conditions are optimized to ensure high yield and purity of the product. The use of catalysts and controlled temperature and pressure conditions are crucial for efficient production .
Chemical Reactions Analysis
Types of Reactions: Hydroxyethyloxamic acid undergoes various chemical reactions, including:
Oxidation: It can be oxidized to form corresponding oxamic acid derivatives.
Reduction: Reduction reactions can convert it into simpler amine derivatives.
Substitution: It can participate in nucleophilic substitution reactions, where the hydroxyethyl group can be replaced by other functional groups.
Common Reagents and Conditions:
Oxidation: Common oxidizing agents include potassium permanganate and hydrogen peroxide.
Reduction: Reducing agents such as lithium aluminum hydride are often used.
Substitution: Nucleophiles like halides or amines can be used under basic or acidic conditions.
Major Products Formed: The major products formed from these reactions depend on the specific reagents and conditions used. For example, oxidation may yield oxamic acid derivatives, while substitution reactions can produce a variety of functionalized oxamic acids .
Scientific Research Applications
Hydroxyethyloxamic acid has a wide range of applications in scientific research:
Chemistry: It is used as a building block in the synthesis of more complex organic molecules.
Medicine: this compound derivatives are explored for their potential as therapeutic agents, particularly in cancer research due to their ability to inhibit specific enzymes.
Industry: It is used in the production of polymers and as a chelating agent in various industrial processes.
Comparison with Similar Compounds
Acetohydroxamic acid: Similar in structure but with an acetyl group instead of a hydroxyethyl group.
Hydroxamic acids: A broader class of compounds that include various derivatives with different substituents.
Uniqueness: Hydroxyethyloxamic acid is unique due to its specific hydroxyethyl substitution, which imparts distinct chemical properties and reactivity compared to other hydroxamic acids. This uniqueness makes it valuable in specific applications, particularly in medicinal chemistry and industrial processes .
Properties
IUPAC Name |
2-(2-hydroxyethylamino)-2-oxoacetic acid | |
---|---|---|
Source | PubChem | |
URL | https://pubchem.ncbi.nlm.nih.gov | |
Description | Data deposited in or computed by PubChem | |
InChI |
InChI=1S/C4H7NO4/c6-2-1-5-3(7)4(8)9/h6H,1-2H2,(H,5,7)(H,8,9) | |
Source | PubChem | |
URL | https://pubchem.ncbi.nlm.nih.gov | |
Description | Data deposited in or computed by PubChem | |
InChI Key |
IYENBEYPOIXIHY-UHFFFAOYSA-N | |
Source | PubChem | |
URL | https://pubchem.ncbi.nlm.nih.gov | |
Description | Data deposited in or computed by PubChem | |
Canonical SMILES |
C(CO)NC(=O)C(=O)O | |
Source | PubChem | |
URL | https://pubchem.ncbi.nlm.nih.gov | |
Description | Data deposited in or computed by PubChem | |
Molecular Formula |
C4H7NO4 | |
Source | PubChem | |
URL | https://pubchem.ncbi.nlm.nih.gov | |
Description | Data deposited in or computed by PubChem | |
DSSTOX Substance ID |
DTXSID40200668 | |
Record name | Hydroxyethyloxamic acid | |
Source | EPA DSSTox | |
URL | https://comptox.epa.gov/dashboard/DTXSID40200668 | |
Description | DSSTox provides a high quality public chemistry resource for supporting improved predictive toxicology. | |
Molecular Weight |
133.10 g/mol | |
Source | PubChem | |
URL | https://pubchem.ncbi.nlm.nih.gov | |
Description | Data deposited in or computed by PubChem | |
CAS No. |
5270-73-5 | |
Record name | Hydroxyethyloxamic acid | |
Source | ChemIDplus | |
URL | https://pubchem.ncbi.nlm.nih.gov/substance/?source=chemidplus&sourceid=0005270735 | |
Description | ChemIDplus is a free, web search system that provides access to the structure and nomenclature authority files used for the identification of chemical substances cited in National Library of Medicine (NLM) databases, including the TOXNET system. | |
Record name | Hydroxyethyloxamic acid | |
Source | EPA DSSTox | |
URL | https://comptox.epa.gov/dashboard/DTXSID40200668 | |
Description | DSSTox provides a high quality public chemistry resource for supporting improved predictive toxicology. | |
Synthesis routes and methods
Procedure details
Retrosynthesis Analysis
AI-Powered Synthesis Planning: Our tool employs the Template_relevance Pistachio, Template_relevance Bkms_metabolic, Template_relevance Pistachio_ringbreaker, Template_relevance Reaxys, Template_relevance Reaxys_biocatalysis model, leveraging a vast database of chemical reactions to predict feasible synthetic routes.
One-Step Synthesis Focus: Specifically designed for one-step synthesis, it provides concise and direct routes for your target compounds, streamlining the synthesis process.
Accurate Predictions: Utilizing the extensive PISTACHIO, BKMS_METABOLIC, PISTACHIO_RINGBREAKER, REAXYS, REAXYS_BIOCATALYSIS database, our tool offers high-accuracy predictions, reflecting the latest in chemical research and data.
Strategy Settings
Precursor scoring | Relevance Heuristic |
---|---|
Min. plausibility | 0.01 |
Model | Template_relevance |
Template Set | Pistachio/Bkms_metabolic/Pistachio_ringbreaker/Reaxys/Reaxys_biocatalysis |
Top-N result to add to graph | 6 |
Feasible Synthetic Routes
Disclaimer and Information on In-Vitro Research Products
Please be aware that all articles and product information presented on BenchChem are intended solely for informational purposes. The products available for purchase on BenchChem are specifically designed for in-vitro studies, which are conducted outside of living organisms. In-vitro studies, derived from the Latin term "in glass," involve experiments performed in controlled laboratory settings using cells or tissues. It is important to note that these products are not categorized as medicines or drugs, and they have not received approval from the FDA for the prevention, treatment, or cure of any medical condition, ailment, or disease. We must emphasize that any form of bodily introduction of these products into humans or animals is strictly prohibited by law. It is essential to adhere to these guidelines to ensure compliance with legal and ethical standards in research and experimentation.