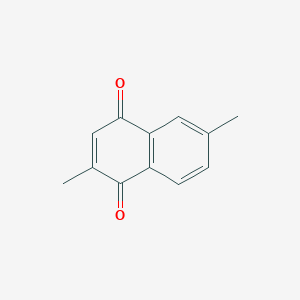
2,6-Dimethylnaphthalene-1,4-dione
- Click on QUICK INQUIRY to receive a quote from our team of experts.
- With the quality product at a COMPETITIVE price, you can focus more on your research.
Description
2,6-Dimethylnaphthalene-1,4-dione, also known as this compound, is a useful research compound. Its molecular formula is C12H10O2 and its molecular weight is 186.21 g/mol. The purity is usually 95%.
The exact mass of the compound this compound is unknown and the complexity rating of the compound is unknown. The compound has been submitted to the National Cancer Institute (NCI) for testing and evaluation and the Cancer Chemotherapy National Service Center (NSC) number is 4267. The storage condition is unknown. Please store according to label instructions upon receipt of goods.
BenchChem offers high-quality this compound suitable for many research applications. Different packaging options are available to accommodate customers' requirements. Please inquire for more information about this compound including the price, delivery time, and more detailed information at info@benchchem.com.
Scientific Research Applications
Medicinal Chemistry
Antimicrobial and Antitumor Activities
Research has demonstrated that naphthoquinones, including derivatives like 2,6-dimethylnaphthalene-1,4-dione, exhibit significant antimicrobial and antitumor properties. These compounds have shown effectiveness against various bacterial strains such as Klebsiella oxytoca and Staphylococcus aureus, with some complexes outperforming traditional antibiotics in vitro . Additionally, studies indicate potential anticancer activities, particularly in targeting resistant cancer cell lines .
Neuroprotective Effects
Recent studies highlight the neuroprotective capabilities of naphthoquinones. They have been found to protect against neurodegenerative diseases by mitigating oxidative stress and inflammation . This suggests that this compound could play a role in developing therapies for conditions like Alzheimer's disease.
Biochemical Tools
Naphthoquinones are also being explored as biochemical tools for non-invasive imaging techniques. Their ability to target specific cellular pathways makes them suitable for detecting pathological areas in tissues affected by diseases such as myocardial infarction .
Material Science
Nanotechnology Applications
In material science, this compound is being investigated for its role in the synthesis of nanoparticles. These nanoparticles can be functionalized for various applications in biosensing and drug delivery systems. The unique properties of naphthoquinones enhance the stability and reactivity of these nanoparticles, making them valuable in biomedical applications .
Photovoltaic Devices
The compound's electronic properties allow it to be used in organic photovoltaic devices. Its ability to act as a light-harvesting material can improve the efficiency of solar cells . Research is ongoing to optimize its performance in these applications.
Environmental Science
Pesticide Development
The chemical structure of this compound presents opportunities for developing new pesticides. Its biological activity can be harnessed to create compounds that effectively target pests while minimizing environmental impact . Studies are underway to evaluate its efficacy and safety in agricultural settings.
Table 1: Biological Activities of this compound Derivatives
Activity Type | Target Organism/Cell Type | IC50 Value (μM) | Reference |
---|---|---|---|
Antibacterial | Klebsiella oxytoca | 12 | |
Antitumor | HCT-116 (PTX-resistant) | 0.25 | |
Neuroprotective | Neuro-2a cells | - | |
Photovoltaic | Organic solar cells | - |
Table 2: Potential Applications in Various Fields
Field | Application Description |
---|---|
Medicinal Chemistry | Antimicrobial agents and anticancer therapies |
Material Science | Nanoparticle synthesis and photovoltaic devices |
Environmental Science | Development of eco-friendly pesticides |
Q & A
Basic Research Questions
Q. What are the standard synthetic routes for 2,6-dimethylnaphthalene-1,4-dione, and how is structural validation performed?
- Methodological Answer : A common approach involves electrophilic substitution reactions using dihydroxynaphthalene derivatives. For example, adamantylation of 1,4-dihydroxynaphthalene in trifluoroacetic acid yields diketone derivatives, as confirmed by 1H and 13C NMR spectroscopy to verify regioselectivity and structural integrity . Solvent choice (e.g., DMF with K2CO3) and reaction monitoring via TLC are critical for optimizing yields .
Q. What safety protocols are essential when handling this compound in laboratory settings?
- Methodological Answer : The compound is classified under acute toxicity (Category 4) for oral, dermal, and inhalation exposure. Researchers must use PPE (gloves, goggles, lab coats), work in fume hoods, and ensure proper ventilation. Emergency measures include immediate rinsing for skin/eye contact and medical consultation for inhalation exposure .
Q. How are the physicochemical properties (e.g., solubility, stability) of this compound determined?
- Methodological Answer : Computational tools predict properties like pKa (2.77 ± 0.50) and density (1.199 g/cm3), while experimental validation involves HPLC for solubility profiling and thermogravimetric analysis (TGA) for thermal stability. NMR and mass spectrometry further confirm molecular weight (184.1940 g/mol) and purity .
Advanced Research Questions
Q. How do reaction conditions influence the regioselectivity of substitutions in this compound derivatives?
- Methodological Answer : Steric and electronic factors dictate regioselectivity. For instance, adamantylation in trifluoroacetic acid favors diketone formation at the 2-position due to acid-catalyzed keto-enol tautomerism. Comparative studies with tert-butyl or amyl alcohols reveal solvent-dependent outcomes, requiring DFT calculations to map energy barriers and transition states .
Q. What computational strategies are used to resolve contradictions in toxicity data across species?
- Methodological Answer : Species-specific metabolic pathways (e.g., cytochrome P450 activity in rodents vs. humans) explain divergent toxicity profiles. In silico models like QSAR and molecular docking predict metabolite interactions, while in vitro assays (e.g., hepatic microsomal studies) validate findings. Cross-referencing toxicogenomic databases ensures robust risk assessment .
Q. How can crystallography and Hirshfeld surface analysis resolve structural ambiguities in synthesized derivatives?
- Methodological Answer : X-ray crystallography provides precise bond lengths and angles, while Hirshfeld analysis quantifies intermolecular interactions (e.g., H···H, O···H contacts). For example, C2-symmetrical bis-(β-enamino-pyran-2,4-dione) derivatives show crystal stability dominated by H···C interactions (15–20% contribution), validated against DFT-computed dipole moments and NMR chemical shifts (R2=0.93–0.94) .
Q. What methodologies address discrepancies in environmental persistence data for polycyclic quinones like this compound?
- Methodological Answer : Accelerated degradation studies under UV/VIS irradiation simulate environmental conditions. LC-MS/MS identifies photolytic byproducts, while QTOF-MS tracks hydroxyl radical formation. Comparative analysis with structurally related compounds (e.g., 1,3-dimethylnaphthalene) clarifies degradation kinetics and half-life variability .
Q. Data Contradiction Analysis
Q. Why do systemic toxicity outcomes vary between inhalation and dermal exposure routes in animal studies?
- Methodological Answer : Inhalation bypasses first-pass metabolism, leading to higher bioavailability and acute respiratory effects (e.g., alveolar damage in rodents). Dermal studies often show delayed systemic effects (e.g., hepatic enzyme induction) due to slower absorption. Meta-analyses of LD50 values and histopathology data reconcile route-specific toxicodynamic profiles .
Q. How can researchers validate conflicting computational predictions of electronic properties for quinone-based compounds?
- Methodological Answer : Hybrid DFT methods (e.g., B3LYP/6-311+G(d,p)) calculate HOMO-LUMO gaps and electrostatic potentials, which are cross-validated with cyclic voltammetry (redox potentials) and UV-Vis spectroscopy (bandgap alignment). Discrepancies often arise from solvent effects or basis set limitations, necessitating experimental calibration .
Properties
CAS No. |
6290-94-4 |
---|---|
Molecular Formula |
C12H10O2 |
Molecular Weight |
186.21 g/mol |
IUPAC Name |
2,6-dimethylnaphthalene-1,4-dione |
InChI |
InChI=1S/C12H10O2/c1-7-3-4-9-10(5-7)11(13)6-8(2)12(9)14/h3-6H,1-2H3 |
InChI Key |
SRLBDWAAORRXIC-UHFFFAOYSA-N |
SMILES |
CC1=CC2=C(C=C1)C(=O)C(=CC2=O)C |
Canonical SMILES |
CC1=CC2=C(C=C1)C(=O)C(=CC2=O)C |
Key on ui other cas no. |
6290-94-4 |
Synonyms |
2,6-dimethyl-1,4-naphthoquinone |
Origin of Product |
United States |
Disclaimer and Information on In-Vitro Research Products
Please be aware that all articles and product information presented on BenchChem are intended solely for informational purposes. The products available for purchase on BenchChem are specifically designed for in-vitro studies, which are conducted outside of living organisms. In-vitro studies, derived from the Latin term "in glass," involve experiments performed in controlled laboratory settings using cells or tissues. It is important to note that these products are not categorized as medicines or drugs, and they have not received approval from the FDA for the prevention, treatment, or cure of any medical condition, ailment, or disease. We must emphasize that any form of bodily introduction of these products into humans or animals is strictly prohibited by law. It is essential to adhere to these guidelines to ensure compliance with legal and ethical standards in research and experimentation.