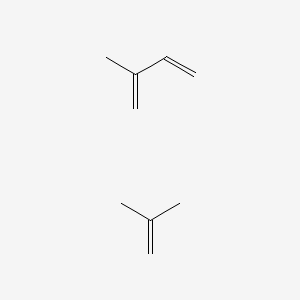
Butyl rubber
Overview
Description
Butyl rubber is a synthetic polymer known for its unique properties and applications. This compound is commonly referred to as this compound, which is a type of synthetic rubber. It is produced by the polymerization of 2-methylpropene (isobutylene) with small amounts of 2-methyl-1,3-butadiene (isoprene). The resulting polymer is highly resistant to chemicals and weathering, making it suitable for various industrial applications .
Preparation Methods
Synthetic Routes and Reaction Conditions
The polymerization of 2-methylpropene with 2-methyl-1,3-butadiene is typically carried out in the presence of a catalyst. One common method involves the use of azobisisobutyronitrile as a radical initiator. The reaction is conducted in a solvent mixture, such as 2,2,4-trimethylpentane and carbon tetrachloride, under an inert nitrogen atmosphere. The reaction mixture is heated to around 75°C, and the polymerization process is allowed to proceed for several hours.
Industrial Production Methods
In industrial settings, the production of butyl rubber involves the copolymerization of isobutylene with isoprene in large reactors. The process is typically carried out at low temperatures to control the polymerization rate and achieve the desired molecular weight distribution. The resulting polymer is then purified and processed into various forms, such as sheets, granules, or coatings .
Chemical Reactions Analysis
Types of Reactions
Butyl rubber undergoes several types of chemical reactions, including:
Oxidation: The polymer can be oxidized to form various oxidation products.
Substitution: The polymer can undergo substitution reactions, where hydrogen atoms are replaced by other functional groups.
Cross-linking: The polymer chains can be cross-linked through vulcanization, which involves the formation of sulfur bridges between polymer chains.
Common Reagents and Conditions
Oxidation: Common oxidizing agents include hydrogen peroxide and ozone.
Substitution: Reagents such as halogens (e.g., chlorine, bromine) are used for substitution reactions.
Cross-linking: Sulfur or sulfur-containing compounds are used in the vulcanization process.
Major Products Formed
Oxidation: Oxidized derivatives of the polymer.
Substitution: Halogenated polymers.
Cross-linking: Vulcanized rubber with enhanced mechanical properties.
Scientific Research Applications
Butyl rubber has a wide range of scientific research applications, including:
Chemistry: Used as a model compound for studying polymerization mechanisms and kinetics.
Biology: Employed in the development of biocompatible materials for medical devices and implants.
Medicine: Utilized in the production of drug delivery systems and medical tubing.
Mechanism of Action
The mechanism by which Butyl rubber exerts its effects involves the formation of a highly stable polymer network. The polymer chains are cross-linked through vulcanization, which enhances the mechanical strength and chemical resistance of the material. The molecular targets and pathways involved in this process include the formation of sulfur bridges between polymer chains, which create a three-dimensional network structure .
Comparison with Similar Compounds
Similar Compounds
1,3-Butadiene, 2-methyl-, homopolymer:
2-Propenoic acid, 2-methyl-, methyl ester, polymer with 1,3-butadiene: A copolymer used in various applications, including adhesives and coatings.
Uniqueness
Butyl rubber is unique due to its high chemical resistance and durability. Unlike other similar compounds, it can be cross-linked through vulcanization to form a highly stable and resilient material. This makes it particularly suitable for applications requiring long-term performance under harsh conditions .
Properties
CAS No. |
68081-82-3 |
---|---|
Molecular Formula |
C9H16 |
Molecular Weight |
124.22 g/mol |
IUPAC Name |
2-methylbuta-1,3-diene;2-methylprop-1-ene |
InChI |
InChI=1S/C5H8.C4H8/c1-4-5(2)3;1-4(2)3/h4H,1-2H2,3H3;1H2,2-3H3 |
InChI Key |
VHOQXEIFYTTXJU-UHFFFAOYSA-N |
SMILES |
CC(=C)C.CC(=C)C=C |
Canonical SMILES |
CC(=C)C.CC(=C)C=C |
68441-14-5 9010-85-9 68081-82-3 |
|
Synonyms |
butyl rubber |
Origin of Product |
United States |
Synthesis routes and methods I
Procedure details
Synthesis routes and methods II
Procedure details
Synthesis routes and methods III
Procedure details
Synthesis routes and methods IV
Procedure details
Retrosynthesis Analysis
AI-Powered Synthesis Planning: Our tool employs the Template_relevance Pistachio, Template_relevance Bkms_metabolic, Template_relevance Pistachio_ringbreaker, Template_relevance Reaxys, Template_relevance Reaxys_biocatalysis model, leveraging a vast database of chemical reactions to predict feasible synthetic routes.
One-Step Synthesis Focus: Specifically designed for one-step synthesis, it provides concise and direct routes for your target compounds, streamlining the synthesis process.
Accurate Predictions: Utilizing the extensive PISTACHIO, BKMS_METABOLIC, PISTACHIO_RINGBREAKER, REAXYS, REAXYS_BIOCATALYSIS database, our tool offers high-accuracy predictions, reflecting the latest in chemical research and data.
Strategy Settings
Precursor scoring | Relevance Heuristic |
---|---|
Min. plausibility | 0.01 |
Model | Template_relevance |
Template Set | Pistachio/Bkms_metabolic/Pistachio_ringbreaker/Reaxys/Reaxys_biocatalysis |
Top-N result to add to graph | 6 |
Feasible Synthetic Routes
Disclaimer and Information on In-Vitro Research Products
Please be aware that all articles and product information presented on BenchChem are intended solely for informational purposes. The products available for purchase on BenchChem are specifically designed for in-vitro studies, which are conducted outside of living organisms. In-vitro studies, derived from the Latin term "in glass," involve experiments performed in controlled laboratory settings using cells or tissues. It is important to note that these products are not categorized as medicines or drugs, and they have not received approval from the FDA for the prevention, treatment, or cure of any medical condition, ailment, or disease. We must emphasize that any form of bodily introduction of these products into humans or animals is strictly prohibited by law. It is essential to adhere to these guidelines to ensure compliance with legal and ethical standards in research and experimentation.