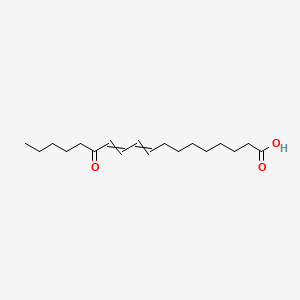
13-oxooctadeca-9,11-dienoic acid
- Click on QUICK INQUIRY to receive a quote from our team of experts.
- With the quality product at a COMPETITIVE price, you can focus more on your research.
Overview
Description
Preparation Methods
Synthetic Routes and Reaction Conditions
The preparation of 13-oxooctadeca-9,11-dienoic acid typically involves the oxidation of linoleic acid. One common method is the use of potassium permanganate (KMnO4) as an oxidizing agent under acidic conditions. The reaction proceeds through the formation of an intermediate diol, which is then further oxidized to form the keto group at the 13th carbon position .
Industrial Production Methods
Industrial production of this compound can be achieved through large-scale oxidation processes. These processes often utilize continuous flow reactors to ensure efficient and consistent production. The use of catalysts, such as manganese dioxide (MnO2), can enhance the reaction rate and yield .
Chemical Reactions Analysis
Types of Reactions
13-oxooctadeca-9,11-dienoic acid undergoes various chemical reactions, including:
Oxidation: Further oxidation can convert this compound into carboxylic acids.
Reduction: Reduction reactions can convert the keto group back to a hydroxyl group, forming 13-hydroxy-9Z,11E-octadecadienoic acid.
Substitution: The keto group can participate in nucleophilic substitution reactions, forming various derivatives .
Common Reagents and Conditions
Oxidation: Potassium permanganate (KMnO4) or chromium trioxide (CrO3) under acidic conditions.
Reduction: Sodium borohydride (NaBH4) or lithium aluminum hydride (LiAlH4) under mild conditions.
Substitution: Grignard reagents (RMgX) or organolithium reagents (RLi) under anhydrous conditions .
Major Products Formed
Oxidation: Carboxylic acids.
Reduction: 13-hydroxy-9Z,11E-octadecadienoic acid.
Substitution: Various alkyl or aryl derivatives depending on the nucleophile used .
Scientific Research Applications
13-oxooctadeca-9,11-dienoic acid has a wide range of scientific research applications:
Chemistry: Used as a precursor for the synthesis of various organic compounds.
Biology: Studied for its role in lipid metabolism and signaling pathways.
Medicine: Investigated for its potential anti-inflammatory and anti-cancer properties.
Industry: Utilized in the production of bio-based materials and as a chemical intermediate in various industrial processes .
Mechanism of Action
The mechanism of action of 13-oxooctadeca-9,11-dienoic acid involves its interaction with specific molecular targets and pathways. It is known to modulate the activity of peroxisome proliferator-activated receptors (PPARs), which are involved in the regulation of lipid metabolism and inflammation. By binding to these receptors, this compound can influence gene expression and cellular responses .
Comparison with Similar Compounds
Similar Compounds
13-HODE (13-hydroxy-9Z,11E-octadecadienoic acid): A hydroxylated derivative of linoleic acid.
9-ketoODE (9-oxo-10E,12Z-octadecadienoic acid): Another oxooctadecadienoic acid with the keto group at the 9th position.
13-DODE (13-dihydroxy-9Z,11E-octadecadienoic acid): A dihydroxylated derivative of linoleic acid .
Uniqueness
13-oxooctadeca-9,11-dienoic acid is unique due to its specific keto group at the 13th position, which imparts distinct chemical and biological properties. This structural feature allows it to participate in unique reactions and interact with specific molecular targets, differentiating it from other similar compounds .
Properties
Molecular Formula |
C18H30O3 |
---|---|
Molecular Weight |
294.4 g/mol |
IUPAC Name |
13-oxooctadeca-9,11-dienoic acid |
InChI |
InChI=1S/C18H30O3/c1-2-3-11-14-17(19)15-12-9-7-5-4-6-8-10-13-16-18(20)21/h7,9,12,15H,2-6,8,10-11,13-14,16H2,1H3,(H,20,21) |
InChI Key |
JHXAZBBVQSRKJR-UHFFFAOYSA-N |
SMILES |
CCCCCC(=O)C=CC=CCCCCCCCC(=O)O |
Canonical SMILES |
CCCCCC(=O)C=CC=CCCCCCCCC(=O)O |
Synonyms |
13-keto-9,11,-octadecadienoic acid 13-KODDA 13-oxo-9,11-octadecadienoic acid 13-OXO-ODE 13-oxooctadecadienoic acid |
Origin of Product |
United States |
Retrosynthesis Analysis
AI-Powered Synthesis Planning: Our tool employs the Template_relevance Pistachio, Template_relevance Bkms_metabolic, Template_relevance Pistachio_ringbreaker, Template_relevance Reaxys, Template_relevance Reaxys_biocatalysis model, leveraging a vast database of chemical reactions to predict feasible synthetic routes.
One-Step Synthesis Focus: Specifically designed for one-step synthesis, it provides concise and direct routes for your target compounds, streamlining the synthesis process.
Accurate Predictions: Utilizing the extensive PISTACHIO, BKMS_METABOLIC, PISTACHIO_RINGBREAKER, REAXYS, REAXYS_BIOCATALYSIS database, our tool offers high-accuracy predictions, reflecting the latest in chemical research and data.
Strategy Settings
Precursor scoring | Relevance Heuristic |
---|---|
Min. plausibility | 0.01 |
Model | Template_relevance |
Template Set | Pistachio/Bkms_metabolic/Pistachio_ringbreaker/Reaxys/Reaxys_biocatalysis |
Top-N result to add to graph | 6 |
Feasible Synthetic Routes
Disclaimer and Information on In-Vitro Research Products
Please be aware that all articles and product information presented on BenchChem are intended solely for informational purposes. The products available for purchase on BenchChem are specifically designed for in-vitro studies, which are conducted outside of living organisms. In-vitro studies, derived from the Latin term "in glass," involve experiments performed in controlled laboratory settings using cells or tissues. It is important to note that these products are not categorized as medicines or drugs, and they have not received approval from the FDA for the prevention, treatment, or cure of any medical condition, ailment, or disease. We must emphasize that any form of bodily introduction of these products into humans or animals is strictly prohibited by law. It is essential to adhere to these guidelines to ensure compliance with legal and ethical standards in research and experimentation.