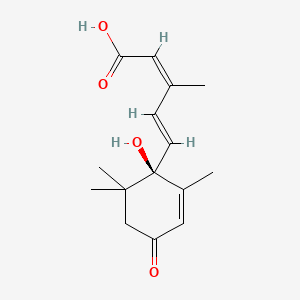
(-)-Abscisic acid
Overview
Description
(-)-Abscisic acid: is a naturally occurring plant hormone that plays a crucial role in various physiological processes, including seed dormancy, germination, and response to environmental stress. It is a sesquiterpenoid, which means it is derived from three isoprene units. This compound is essential for plants to adapt to changing environmental conditions, such as drought and high salinity.
Preparation Methods
Synthetic Routes and Reaction Conditions:
Oxidation of Zeaxanthin: One common method involves the oxidation of zeaxanthin, a carotenoid, using potassium permanganate or other oxidizing agents. This process yields violaxanthin, which is further oxidized to produce (-)-Abscisic acid.
Biosynthetic Pathway: In plants, this compound is synthesized from carotenoids through a series of enzymatic reactions. The key intermediate in this pathway is xanthoxin, which is converted to this compound via oxidation and isomerization reactions.
Industrial Production Methods: Industrial production of this compound typically involves the chemical synthesis route due to its efficiency and scalability. The process starts with readily available carotenoids and involves multiple steps of oxidation and purification to obtain the final product.
Chemical Reactions Analysis
Types of Reactions:
Oxidation: (-)-Abscisic acid can undergo oxidation reactions to form phaseic acid and dihydrophaseic acid.
Reduction: Reduction of this compound can yield abscisyl alcohol.
Hydrolysis: Hydrolysis of this compound esters can produce the free acid form.
Common Reagents and Conditions:
Oxidizing Agents: Potassium permanganate, hydrogen peroxide.
Reducing Agents: Sodium borohydride, lithium aluminum hydride.
Hydrolysis Conditions: Acidic or basic aqueous solutions.
Major Products Formed:
Phaseic Acid: Formed through oxidation.
Abscisyl Alcohol: Formed through reduction.
Free this compound: Formed through hydrolysis of esters.
Scientific Research Applications
Chemistry:
Synthesis of Derivatives: (-)-Abscisic acid is used as a precursor for synthesizing various derivatives for research purposes.
Biology:
Plant Physiology: It is extensively studied for its role in regulating plant growth, development, and stress responses.
Signal Transduction: Research focuses on understanding how this compound signals are perceived and transduced within plant cells.
Medicine:
Therapeutic Potential: Studies are exploring its potential use in treating diseases related to oxidative stress and inflammation.
Industry:
Agriculture: this compound is used to enhance crop resilience to environmental stressors, improve seed germination rates, and regulate fruit ripening.
Mechanism of Action
Molecular Targets and Pathways:
Signal Transduction Pathway: Upon binding to its receptors, (-)-Abscisic acid activates a signaling cascade involving protein phosphatases (PP2Cs) and kinases (SnRK2s), leading to the regulation of gene expression and physiological responses.
Stress Response: This signaling pathway helps plants respond to abiotic stress by closing stomata, reducing water loss, and activating stress-responsive genes.
Comparison with Similar Compounds
Gibberellic Acid: Another plant hormone involved in growth regulation but promotes growth rather than inhibiting it.
Indole-3-Acetic Acid: A plant hormone that promotes cell elongation and division.
Jasmonic Acid: Involved in plant defense responses and stress signaling.
Uniqueness of (-)-Abscisic acid:
Stress Response: Unlike other plant hormones, this compound is primarily involved in stress responses, particularly drought and salinity stress.
Seed Dormancy: It plays a unique role in inducing and maintaining seed dormancy, which is not a primary function of other plant hormones.
Properties
IUPAC Name |
(2Z,4E)-5-[(1R)-1-hydroxy-2,6,6-trimethyl-4-oxocyclohex-2-en-1-yl]-3-methylpenta-2,4-dienoic acid | |
---|---|---|
Source | PubChem | |
URL | https://pubchem.ncbi.nlm.nih.gov | |
Description | Data deposited in or computed by PubChem | |
InChI |
InChI=1S/C15H20O4/c1-10(7-13(17)18)5-6-15(19)11(2)8-12(16)9-14(15,3)4/h5-8,19H,9H2,1-4H3,(H,17,18)/b6-5+,10-7-/t15-/m0/s1 | |
Source | PubChem | |
URL | https://pubchem.ncbi.nlm.nih.gov | |
Description | Data deposited in or computed by PubChem | |
InChI Key |
JLIDBLDQVAYHNE-QHFMCZIYSA-N | |
Source | PubChem | |
URL | https://pubchem.ncbi.nlm.nih.gov | |
Description | Data deposited in or computed by PubChem | |
Canonical SMILES |
CC1=CC(=O)CC(C1(C=CC(=CC(=O)O)C)O)(C)C | |
Source | PubChem | |
URL | https://pubchem.ncbi.nlm.nih.gov | |
Description | Data deposited in or computed by PubChem | |
Isomeric SMILES |
CC1=CC(=O)CC([C@@]1(/C=C/C(=C\C(=O)O)/C)O)(C)C | |
Source | PubChem | |
URL | https://pubchem.ncbi.nlm.nih.gov | |
Description | Data deposited in or computed by PubChem | |
Molecular Formula |
C15H20O4 | |
Source | PubChem | |
URL | https://pubchem.ncbi.nlm.nih.gov | |
Description | Data deposited in or computed by PubChem | |
DSSTOX Substance ID |
DTXSID701033205 | |
Record name | (2Z,4E)-5-[(1R)-1-Hydroxy-2,6,6-trimethyl-4-oxo-2-cyclohexen-1-yl]-3-methyl-2,4-pentadienoic acid | |
Source | EPA DSSTox | |
URL | https://comptox.epa.gov/dashboard/DTXSID701033205 | |
Description | DSSTox provides a high quality public chemistry resource for supporting improved predictive toxicology. | |
Molecular Weight |
264.32 g/mol | |
Source | PubChem | |
URL | https://pubchem.ncbi.nlm.nih.gov | |
Description | Data deposited in or computed by PubChem | |
CAS No. |
14398-53-9, 21293-29-8 | |
Record name | Abscisic acid, (-)-(2Z,4E)- | |
Source | ChemIDplus | |
URL | https://pubchem.ncbi.nlm.nih.gov/substance/?source=chemidplus&sourceid=0014398539 | |
Description | ChemIDplus is a free, web search system that provides access to the structure and nomenclature authority files used for the identification of chemical substances cited in National Library of Medicine (NLM) databases, including the TOXNET system. | |
Record name | (2Z,4E)-5-[(1R)-1-Hydroxy-2,6,6-trimethyl-4-oxo-2-cyclohexen-1-yl]-3-methyl-2,4-pentadienoic acid | |
Source | EPA DSSTox | |
URL | https://comptox.epa.gov/dashboard/DTXSID701033205 | |
Description | DSSTox provides a high quality public chemistry resource for supporting improved predictive toxicology. | |
Record name | [S-(Z,E)]-5-(1-hydroxy-2,6,6-trimethyl-4-oxocyclohex-2-en-1-yl)-3-methylpenta-2,4-dienoic acid | |
Source | European Chemicals Agency (ECHA) | |
URL | https://echa.europa.eu/substance-information/-/substanceinfo/100.040.275 | |
Description | The European Chemicals Agency (ECHA) is an agency of the European Union which is the driving force among regulatory authorities in implementing the EU's groundbreaking chemicals legislation for the benefit of human health and the environment as well as for innovation and competitiveness. | |
Explanation | Use of the information, documents and data from the ECHA website is subject to the terms and conditions of this Legal Notice, and subject to other binding limitations provided for under applicable law, the information, documents and data made available on the ECHA website may be reproduced, distributed and/or used, totally or in part, for non-commercial purposes provided that ECHA is acknowledged as the source: "Source: European Chemicals Agency, http://echa.europa.eu/". Such acknowledgement must be included in each copy of the material. ECHA permits and encourages organisations and individuals to create links to the ECHA website under the following cumulative conditions: Links can only be made to webpages that provide a link to the Legal Notice page. | |
Record name | ABSCISIC ACID, (-)-(2Z,4E)- | |
Source | FDA Global Substance Registration System (GSRS) | |
URL | https://gsrs.ncats.nih.gov/ginas/app/beta/substances/4F7961S98F | |
Description | The FDA Global Substance Registration System (GSRS) enables the efficient and accurate exchange of information on what substances are in regulated products. Instead of relying on names, which vary across regulatory domains, countries, and regions, the GSRS knowledge base makes it possible for substances to be defined by standardized, scientific descriptions. | |
Explanation | Unless otherwise noted, the contents of the FDA website (www.fda.gov), both text and graphics, are not copyrighted. They are in the public domain and may be republished, reprinted and otherwise used freely by anyone without the need to obtain permission from FDA. Credit to the U.S. Food and Drug Administration as the source is appreciated but not required. | |
Retrosynthesis Analysis
AI-Powered Synthesis Planning: Our tool employs the Template_relevance Pistachio, Template_relevance Bkms_metabolic, Template_relevance Pistachio_ringbreaker, Template_relevance Reaxys, Template_relevance Reaxys_biocatalysis model, leveraging a vast database of chemical reactions to predict feasible synthetic routes.
One-Step Synthesis Focus: Specifically designed for one-step synthesis, it provides concise and direct routes for your target compounds, streamlining the synthesis process.
Accurate Predictions: Utilizing the extensive PISTACHIO, BKMS_METABOLIC, PISTACHIO_RINGBREAKER, REAXYS, REAXYS_BIOCATALYSIS database, our tool offers high-accuracy predictions, reflecting the latest in chemical research and data.
Strategy Settings
Precursor scoring | Relevance Heuristic |
---|---|
Min. plausibility | 0.01 |
Model | Template_relevance |
Template Set | Pistachio/Bkms_metabolic/Pistachio_ringbreaker/Reaxys/Reaxys_biocatalysis |
Top-N result to add to graph | 6 |
Feasible Synthetic Routes
Q1: How does abscisic acid interact with its target, and what are the downstream effects of this interaction?
A1: Abscisic acid interacts with a family of soluble PYR/PYL/RCAR receptors. [] This interaction inactivates type 2C protein phosphatases (PP2Cs), which act as negative regulators of ABA signaling. [] This inactivation allows the activation of downstream Snf1-related protein kinases (SnRK2s), triggering a phosphorylation cascade that ultimately leads to ABA-mediated responses, such as stomatal closure and gene expression. []
Q2: Can you elaborate on the role of protein phosphorylation in abscisic acid signaling?
A2: Abscisic acid signaling involves a complex interplay of protein kinases and phosphatases. While PP2Cs act as negative regulators, SnRK2s, particularly SnRK2.2, -2.3, and -2.6, are positive regulators of ABA signaling. [] These kinases are activated through autophosphorylation upon ABA-induced PP2C inhibition. [] This phosphorylation cascade ultimately leads to the activation of downstream transcription factors, such as ABF2, which regulate the expression of ABA-responsive genes. []
Q3: Does abscisic acid interact with other plant hormones?
A3: Yes, abscisic acid interacts with other plant hormones, notably cytokinins. Studies suggest that cytokinins can antagonize abscisic acid effects by enhancing its metabolism, promoting the conversion of abscisic acid to phaseic acid, and potentially suppressing xanthoxal oxidation. []
Q4: What is the molecular formula, weight, and available spectroscopic data for abscisic acid?
A4: Abscisic acid has the molecular formula C15H20O4 and a molecular weight of 264.32 g/mol. Spectroscopic data, such as IR and ORD, have been used to confirm the purity and identity of abscisic acid isolated from plant sources. [, ]
Q5: How stable is abscisic acid under different environmental conditions?
A5: Abscisic acid can be metabolized by plant tissues into various compounds, including phaseic acid, dihydrophaseic acid, and other polar metabolites. [, ] This metabolism can vary depending on the plant species, tissue type, and environmental conditions. For instance, cold stratification can lead to increased abscisic acid metabolism in dormant ash seeds. []
Q6: Are there any specific methods or formulations to enhance the stability or bioavailability of abscisic acid for research or application purposes?
A6: While the provided research does not delve into specific formulation strategies for abscisic acid, studies often utilize solvents like aqueous acetone with Tween 20 for exogenous application. [] Further research is needed to explore targeted delivery methods and formulations that optimize its stability and bioavailability.
Q7: What are the primary physiological effects of abscisic acid in plants?
A7: Abscisic acid plays a crucial role in regulating plant responses to various stresses, including drought, salinity, and extreme temperatures. [] One of its primary functions is regulating stomatal closure to reduce water loss during drought stress. [, ] It also influences seed dormancy and germination, often acting antagonistically with gibberellins. [, , ]
Q8: How does abscisic acid contribute to plant adaptation to drought stress?
A8: Abscisic acid levels increase in response to drought stress, triggering a cascade of events that help plants conserve water and survive under low water conditions. [, ] This includes inducing stomatal closure to reduce transpiration, promoting root growth to enhance water uptake, and regulating the expression of stress-responsive genes involved in osmotic adjustment and detoxification of reactive oxygen species. [, , ]
Q9: What are some key genes involved in abscisic acid biosynthesis and signaling?
A10: Several genes play crucial roles in abscisic acid biosynthesis and signaling pathways. NCED genes are involved in the early steps of ABA biosynthesis. [, ] In ABA signaling, genes like ABI1 and ABI2 encode protein phosphatases that negatively regulate the pathway. [] Transcription factors like ABF2 and MYB96 are positive regulators, controlling the expression of downstream ABA-responsive genes. [, ]
Q10: What are some valuable research tools and techniques used to study abscisic acid?
A11: Researchers employ various techniques to study abscisic acid. These include: * Gas chromatography-mass spectrometry (GC-MS) for identifying and quantifying abscisic acid and its metabolites in plant tissues. [] * Liquid chromatography-mass spectrometry (LC-MS) for analyzing abscisic acid levels and investigating its metabolic pathways. [] * Gene expression analysis (e.g., real-time PCR) to study the expression patterns of ABA-related genes under different conditions. [] * Genetic manipulation (e.g., overexpression, RNA interference) to investigate the functions of specific genes involved in abscisic acid biosynthesis and signaling. [, , ]
Disclaimer and Information on In-Vitro Research Products
Please be aware that all articles and product information presented on BenchChem are intended solely for informational purposes. The products available for purchase on BenchChem are specifically designed for in-vitro studies, which are conducted outside of living organisms. In-vitro studies, derived from the Latin term "in glass," involve experiments performed in controlled laboratory settings using cells or tissues. It is important to note that these products are not categorized as medicines or drugs, and they have not received approval from the FDA for the prevention, treatment, or cure of any medical condition, ailment, or disease. We must emphasize that any form of bodily introduction of these products into humans or animals is strictly prohibited by law. It is essential to adhere to these guidelines to ensure compliance with legal and ethical standards in research and experimentation.