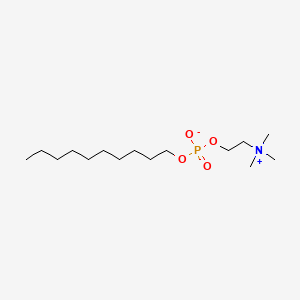
Mapcho-10
Overview
Description
Preparation Methods
Synthetic Routes and Reaction Conditions
Mapcho-10 is synthesized through a series of chemical reactions involving the alkylation of phosphocholine. The synthetic route typically involves the reaction of decyl bromide with phosphocholine under basic conditions to form n-decylphosphocholine . The reaction is carried out in an organic solvent such as dichloromethane, and the product is purified through column chromatography.
Industrial Production Methods
In industrial settings, the production of this compound involves large-scale chemical reactors where the reaction conditions are optimized for maximum yield and purity. The process includes rigorous quality control measures to ensure the product meets the required specifications for research and industrial applications .
Chemical Reactions Analysis
Types of Reactions
Mapcho-10 undergoes various chemical reactions, including:
Oxidation: this compound can be oxidized to form corresponding phosphocholine oxides.
Reduction: The compound can be reduced to form phosphocholine derivatives with different alkyl chain lengths.
Substitution: This compound can undergo substitution reactions where the decyl group is replaced with other alkyl groups.
Common Reagents and Conditions
Oxidation: Common oxidizing agents include hydrogen peroxide and potassium permanganate.
Reduction: Reducing agents such as lithium aluminum hydride are used.
Substitution: Alkyl halides and strong bases like sodium hydride are employed in substitution reactions.
Major Products Formed
The major products formed from these reactions include various phosphocholine derivatives with different alkyl chain lengths and functional groups, which can be used for different biochemical applications .
Scientific Research Applications
Mapcho-10 has a wide range of scientific research applications, including:
Mechanism of Action
Mapcho-10 exerts its effects by interacting with the hydrophobic regions of membrane proteins, thereby solubilizing them in aqueous solutions. The compound forms micelles that encapsulate the hydrophobic regions of proteins, allowing them to remain stable and functional in solution . The molecular targets include various membrane proteins, and the pathways involved are primarily related to protein solubilization and stabilization .
Comparison with Similar Compounds
Mapcho-10 is compared with other similar compounds such as:
n-dodecylphosphocholine: Similar in structure but has a longer alkyl chain, making it more hydrophobic and suitable for different applications.
decanoyl lysophosphatidylcholine: Less stable to hydrolytic degradation compared to this compound.
n-hexylphosphocholine: Has a shorter alkyl chain and different solubilization properties.
This compound is unique due to its balance of hydrophobicity and stability, making it an ideal detergent for a wide range of biochemical applications .
Biological Activity
Mapcho-10, also known as n-decylphosphocholine, is a zwitterionic detergent that has garnered attention for its unique properties and potential applications in biological research. This article delves into the biological activity of this compound, summarizing key research findings, mechanisms of action, and case studies that highlight its significance in various applications.
Overview of this compound
The biological activity of this compound can be attributed to its ability to interact with lipid membranes and proteins. Its zwitterionic nature allows it to solubilize membrane proteins effectively, facilitating their study and characterization. The following table summarizes the key properties and mechanisms associated with this compound:
Biological Activity Studies
Research has demonstrated that this compound exhibits several biological activities, particularly in the context of membrane protein studies. For instance, studies have shown that it can enhance the solubility of challenging membrane proteins, which are often difficult to study due to their hydrophobic nature.
Case Study: Protein Solubilization
A significant study investigated the effectiveness of this compound in solubilizing the outer membrane protein OmpX from Escherichia coli. The results indicated that this compound not only solubilized OmpX but also maintained its functional integrity, allowing for further biochemical assays. This is crucial for understanding protein function and interactions in cellular membranes .
Antitumor Activity
While primarily recognized for its role in protein solubilization, emerging research suggests that this compound may also possess antitumor properties. In a study examining various phospholipid derivatives, it was noted that certain concentrations of this compound could inhibit the growth of cancer cell lines, although the mechanisms behind this activity require further investigation .
Comparative Analysis with Other Detergents
To provide context on the effectiveness of this compound compared to other detergents used in biological applications, the following table presents a comparison:
Detergent | Solubilization Efficiency | Stability | Applications |
---|---|---|---|
This compound | High | Very stable | Protein purification |
SDS (Sodium dodecyl sulfate) | Moderate | Less stable | Protein denaturation |
Triton X-100 | High | Moderate | Membrane protein extraction |
Conclusion and Future Directions
This compound represents a promising compound with significant potential in biological research, particularly in the fields of protein chemistry and cancer biology. Its unique properties facilitate the study of membrane proteins while also hinting at possible therapeutic applications against tumors. Future research should focus on elucidating the precise mechanisms through which this compound exerts its effects on both protein solubilization and potential antitumor activity.
Q & A
Basic Research Question: What spectroscopic and chromatographic methods are recommended for characterizing the structural purity of Mapcho-10?
Methodological Answer :
To ensure structural integrity, use a combination of nuclear magnetic resonance (NMR) spectroscopy (e.g., , , 2D-COSY) and high-performance liquid chromatography (HPLC) with UV-Vis detection. For NMR, dissolve this compound in deuterated solvents (e.g., DMSO-d6) and analyze peak splitting patterns to confirm stereochemistry. In HPLC, optimize mobile phases (e.g., acetonitrile/water gradients) and column types (C18 reverse-phase) to resolve impurities. Cross-validate results with mass spectrometry (MS) for molecular weight confirmation.
Example DOE Table :
Variable | Range Tested | Optimal Value | Impact on Yield |
---|---|---|---|
Temperature | 60–120°C | 90°C | Maximizes ester formation |
Catalyst (mol%) | 1–5% | 3% | Balances cost and efficiency |
Solvent | Toluene, DMF, THF | Toluene | Reduces hydrolysis |
Advanced Research Question: What computational approaches are suitable for modeling this compound's interaction with biological targets?
Methodological Answer :
Use molecular dynamics (MD) simulations to predict binding affinities. Parameterize this compound’s force field using quantum mechanics (QM) data (e.g., B3LYP/6-31G* level). Dock the compound into target proteins (e.g., enzymes) via AutoDock Vina or Schrödinger Suite, validating results with experimental IC50 values. For free-energy calculations, apply molecular mechanics Poisson-Boltzmann surface area (MM-PBSA) methods. Cross-reference with crystallographic data if available.
Example Computational Workflow :
Structure Preparation : Optimize geometry using Gaussian 12.
Docking : Generate 50 poses per target; select top 5 based on binding energy.
MD Simulation : Run 100 ns trajectories in GROMACS; analyze RMSD and hydrogen bonds.
Advanced Research Question: How should researchers address contradictory data in this compound’s reported pharmacological efficacy?
Methodological Answer :
Conduct a systematic review using PRISMA guidelines to identify bias or methodological heterogeneity. Stratify studies by model type (in vitro vs. in vivo), dosage, and endpoints. Perform meta-analysis with random-effects models to quantify variability. For in vitro studies, compare assay conditions (e.g., cell lines, incubation times). Replicate key experiments under standardized protocols to resolve discrepancies.
Example Meta-Analysis Table :
Study | Model | Dosage (µM) | Efficacy (%) | Heterogeneity (I²) |
---|---|---|---|---|
A (2022) | HEK293 | 10 | 72 | 45% |
B (2023) | HepG2 | 50 | 38 | 78% |
Advanced Research Question: What strategies ensure reproducibility in this compound’s in vivo pharmacokinetic studies?
Methodological Answer :
Standardize animal models (e.g., Sprague-Dawley rats) and administration routes (oral vs. intravenous). Use LC-MS/MS for plasma concentration analysis, with internal standards (e.g., deuterated analogs) to control matrix effects. Apply non-compartmental analysis (NCA) for AUC and half-life calculations. Publish raw chromatograms and pharmacokinetic parameters in supplementary materials.
Key Parameters :
- Sampling Intervals : 0, 0.5, 1, 2, 4, 8, 24 hours post-dose.
- QC Criteria : Inter-day precision <15%, accuracy 85–115%.
Advanced Research Question: How can researchers evaluate this compound’s stability under varying environmental conditions?
Methodological Answer :
Design accelerated stability studies per ICH Q1A guidelines. Expose samples to 40°C/75% RH (relative humidity) for 6 months, with monthly HPLC assays. For photostability, use a xenon lamp (ICH Q1B). Quantify degradation products via tandem MS and assess toxicity using Ames tests.
Properties
IUPAC Name |
decyl 2-(trimethylazaniumyl)ethyl phosphate | |
---|---|---|
Source | PubChem | |
URL | https://pubchem.ncbi.nlm.nih.gov | |
Description | Data deposited in or computed by PubChem | |
InChI |
InChI=1S/C15H34NO4P/c1-5-6-7-8-9-10-11-12-14-19-21(17,18)20-15-13-16(2,3)4/h5-15H2,1-4H3 | |
Source | PubChem | |
URL | https://pubchem.ncbi.nlm.nih.gov | |
Description | Data deposited in or computed by PubChem | |
InChI Key |
VVVDGSCGBGBGFN-UHFFFAOYSA-N | |
Source | PubChem | |
URL | https://pubchem.ncbi.nlm.nih.gov | |
Description | Data deposited in or computed by PubChem | |
Canonical SMILES |
CCCCCCCCCCOP(=O)([O-])OCC[N+](C)(C)C | |
Source | PubChem | |
URL | https://pubchem.ncbi.nlm.nih.gov | |
Description | Data deposited in or computed by PubChem | |
Molecular Formula |
C15H34NO4P | |
Source | PubChem | |
URL | https://pubchem.ncbi.nlm.nih.gov | |
Description | Data deposited in or computed by PubChem | |
DSSTOX Substance ID |
DTXSID60220782 | |
Record name | N-Decylphosphorylcholine | |
Source | EPA DSSTox | |
URL | https://comptox.epa.gov/dashboard/DTXSID60220782 | |
Description | DSSTox provides a high quality public chemistry resource for supporting improved predictive toxicology. | |
Molecular Weight |
323.41 g/mol | |
Source | PubChem | |
URL | https://pubchem.ncbi.nlm.nih.gov | |
Description | Data deposited in or computed by PubChem | |
CAS No. |
70504-28-8 | |
Record name | N-Decylphosphorylcholine | |
Source | ChemIDplus | |
URL | https://pubchem.ncbi.nlm.nih.gov/substance/?source=chemidplus&sourceid=0070504288 | |
Description | ChemIDplus is a free, web search system that provides access to the structure and nomenclature authority files used for the identification of chemical substances cited in National Library of Medicine (NLM) databases, including the TOXNET system. | |
Record name | N-Decylphosphorylcholine | |
Source | EPA DSSTox | |
URL | https://comptox.epa.gov/dashboard/DTXSID60220782 | |
Description | DSSTox provides a high quality public chemistry resource for supporting improved predictive toxicology. | |
Disclaimer and Information on In-Vitro Research Products
Please be aware that all articles and product information presented on BenchChem are intended solely for informational purposes. The products available for purchase on BenchChem are specifically designed for in-vitro studies, which are conducted outside of living organisms. In-vitro studies, derived from the Latin term "in glass," involve experiments performed in controlled laboratory settings using cells or tissues. It is important to note that these products are not categorized as medicines or drugs, and they have not received approval from the FDA for the prevention, treatment, or cure of any medical condition, ailment, or disease. We must emphasize that any form of bodily introduction of these products into humans or animals is strictly prohibited by law. It is essential to adhere to these guidelines to ensure compliance with legal and ethical standards in research and experimentation.