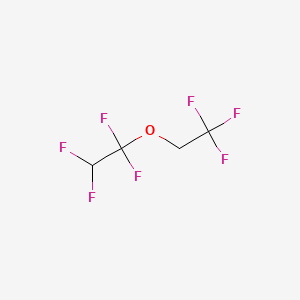
1,1,2,2-Tetrafluoroethyl 2,2,2-trifluoroethyl ether
Overview
Description
1,1,2,2-Tetrafluoroethyl 2,2,2-trifluoroethyl ether (TFTFE, CAS 406-78-0), also known as HFE-347, is a fluorinated ether with the molecular formula C₄H₃F₇O and a molecular weight of 200.05 g/mol . It is characterized by a boiling point of 56°C and a density of 1.479 g/cm³ . TFTFE is widely utilized as a solvent and diluent in advanced battery electrolytes due to its low viscosity, high thermal stability, and non-flammability . Its fluorinated structure enables weak intermolecular interactions, making it effective in dissolving lithium salts and enhancing ionic conductivity in lithium-sulfur (Li-S) and lithium-ion batteries (LIBs) .
Preparation Methods
Synthesis via Catalytic Reaction Systems
The most extensively documented preparation method involves the catalytic reaction of tetrafluoroethylene (C₂F₄) with 2,2,2-trifluoroethanol (CF₃CH₂OH). This approach, detailed in patent CN105061162A, utilizes a composite catalyst system to achieve high yields and purity .
Reaction Mechanism and Stoichiometry
The reaction proceeds via nucleophilic substitution, where the hydroxyl group of trifluoroethanol attacks the electron-deficient tetrafluoroethylene. The composite catalyst facilitates this interaction by stabilizing transition states and minimizing side reactions. The molar ratio of tetrafluoroethylene to trifluoroethanol is critical, with optimal performance observed at 1:1.2 to 1:1.5 . Excess trifluoroethanol ensures complete conversion of tetrafluoroethylene, reducing the formation of oligomeric byproducts.
Key Reaction:
Hydrogen fluoride (HF) is generated as a byproduct, necessitating neutralization protocols during purification .
Catalyst Composition and Optimization
The composite catalyst comprises two primary components:
-
Anion exchange resin (e.g., DOWEX™ MARATHON™): Provides active sites for proton transfer.
-
Ethylenediaminetetraacetic acid (EDTA) disodium salt : Chelates metal impurities, enhancing catalyst longevity .
Table 1: Catalyst Performance Metrics
Catalyst Ratio (Resin:EDTA) | Yield (%) | Purity (%) | Byproducts (%) |
---|---|---|---|
1:0 | 85 | 98.0 | 2.5 |
3:1 | 92 | 99.5 | 0.8 |
2:1 | 90 | 99.2 | 1.1 |
Data from patent examples demonstrate that a 3:1 resin-to-EDTA ratio maximizes yield (92%) and purity (99.5%) while minimizing byproducts .
Reaction Conditions
-
Temperature : 50–80°C (optimal at 65°C)
-
Time : 2–4 hours
-
Pressure : Ambient (exothermic reaction controlled via cooling)
Higher temperatures accelerate reaction kinetics but risk thermal decomposition of the ether product. The patent notes that maintaining the reaction at 65°C for 3 hours achieves a balance between speed and stability .
Purification and Isolation Techniques
Post-synthesis purification is critical to achieving industrial-grade purity. The crude product undergoes sequential steps:
Neutralization and Filtration
Residual HF is neutralized with aqueous sodium bicarbonate, forming insoluble sodium fluoride (NaF). Filtration removes solid NaF and spent catalyst particles .
Distillation Under Reduced Pressure
The filtrate is distilled at 50°C under 20 kPa to isolate the ether. This step separates low-boiling impurities (e.g., unreacted trifluoroethanol) and ensures a purity exceeding 99% .
Table 2: Distillation Parameters
Pressure (kPa) | Temperature (°C) | Purity (%) | Recovery (%) |
---|---|---|---|
20 | 50 | 99.5 | 95 |
30 | 55 | 98.8 | 97 |
Industrial-Scale Production Challenges
Catalyst Recovery and Reuse
While the anion exchange resin is reusable after regeneration, EDTA components degrade over cycles. Patent data indicate a 5% yield drop after three batches, prompting ongoing research into more durable chelators .
Scalability of Distillation
Maintaining high vacuum across large-scale distillation columns remains technically challenging. Alternatives like molecular sieve adsorption are under investigation .
Chemical Reactions Analysis
1,1,2,2-Tetrafluoroethyl 2,2,2-trifluoroethyl ether undergoes various chemical reactions, including:
Oxidation: This compound can be oxidized under specific conditions, leading to the formation of various oxidation products.
Reduction: Reduction reactions can also occur, although they are less common.
Substitution: The ether group can participate in substitution reactions, especially in the presence of strong nucleophiles.
Common reagents used in these reactions include oxidizing agents like potassium permanganate and reducing agents such as lithium aluminum hydride . The major products formed depend on the specific reaction conditions and reagents used .
Scientific Research Applications
Scientific Research Applications
-
Chemical Solvent
- Description: Acts as an environmentally friendly solvent in various chemical processes.
- Applications: Used in organic synthesis and as a medium for chemical reactions due to its stability and low viscosity.
-
Biological Assays
- Description: Utilized in biological experiments to study enzyme interactions and cellular effects.
- Applications: Its ability to influence enzyme activity makes it valuable for biochemical assays and drug discovery.
-
Battery Technologies
- Description: Serves as a co-solvent and additive in lithium-ion and lithium-sulfur batteries.
- Mechanism of Action: Enhances ionic conduction and suppresses dendrite formation during battery cycling.
- Case Study: A study showed that adding 1,1,2,2-tetrafluoroethyl 2,2,2-trifluoroethyl ether to a solvate ionic liquid electrolyte improved the cycle and rate capability of lithium-sulfur cells significantly .
-
Electrolyte Systems
- Description: Functions as a key component in electrolyte formulations for advanced battery systems.
- Benefits: Its unique properties help stabilize the electrolyte interface and enhance overall battery performance.
Case Study 1: Lithium-Sulfur Batteries
Research indicates that incorporating this compound into lithium-sulfur battery systems enhances ionic conductivity and cycle stability. The compound facilitates the formation of a stable interphase that prevents oxidation and metal dissolution while allowing efficient ion transport .
Case Study 2: Enzyme Activity Modulation
In biological assays involving various enzymes and proteins, this compound has been shown to stabilize enzyme activity under specific conditions. Studies reveal that it can modulate cellular responses by influencing metabolic pathways through its interactions with biomolecules .
Mechanism of Action
The mechanism by which 1,1,2,2-tetrafluoroethyl 2,2,2-trifluoroethyl ether exerts its effects is primarily related to its chemical structure. The fluorinated ether group provides high electrochemical stability and low reactivity, making it an ideal solvent and diluent in various applications . In battery systems, it helps to suppress dendrite formation and improve ionic conduction, enhancing the overall performance and longevity of the batteries .
Comparison with Similar Compounds
Comparison with Similar Fluorinated Ethers
Structural and Physicochemical Properties
The table below compares TFTFE with structurally analogous fluorinated ethers:
Key Observations :
- Boiling Points : TFTFE (56°C) has a higher boiling point than BTFE (25°C) but lower than TTE (93.2°C), making it suitable for moderate-temperature applications .
- Density : TFTFE’s density (1.479 g/cm³) is lower than TTE (1.536 g/cm³), reducing electrolyte weight in battery systems .
- Fluorine Content : TTE and F-EPE contain more fluorine atoms, enhancing their flame-retardant properties, while TFTFE balances fluorine content with molecular simplicity .
Electrochemical Performance in Batteries
Ionic Conductivity and Cycle Stability
- TFTFE : In solvate ionic liquids (SILs), TFTFE improves Li-S battery performance by enhancing ionic conductivity (up to 2.5 mS/cm ) and cycle stability (80% capacity retention after 100 cycles) . It acts as a diluent, reducing viscosity without disrupting Li⁺ solvation .
- BTFE : Used in localized high-concentration electrolytes (LHCEs), BTFE lowers viscosity but may compromise ionic conductivity at high rates due to weaker Li⁺ coordination .
- TTE: Exhibits superior high-voltage stability (>4.5 V) and forms robust LiF-rich solid-electrolyte interphases (SEIs) on silicon anodes, outperforming TFTFE in LIBs with silicon-graphite composites .
SEI Formation
- TFTFE : Promotes LiF-rich SEIs via fluorine donation, though less effectively than TTE .
- TTE: Generates SEIs with higher LiF content (40–50 atomic%), critical for preventing anode cracking in silicon-based LIBs .
Cost and Commercial Availability
Biological Activity
1,1,2,2-Tetrafluoroethyl 2,2,2-trifluoroethyl ether (HFE-347pc-f), with the molecular formula C4H3F7O and a molecular weight of 200.05 g/mol, is a fluorinated ether compound that has garnered attention for its potential biological applications and interactions. This article delves into its biochemical properties, cellular effects, and relevant case studies to provide a comprehensive overview of its biological activity.
- Molecular Formula: C4H3F7O
- CAS Number: 406-78-0
- Molar Mass: 200.05 g/mol
- Density: 1.487 g/cm³
- Boiling Point: 50 °C
- Refractive Index: 1.276
HFE-347pc-f is recognized for its role in various biochemical reactions. It interacts with enzymes and proteins, influencing their activity and stability. Notably, it forms a highly fluorinated interphase that can inhibit oxidation and transition metal dissolution in lithium-metal batteries, demonstrating its utility in energy storage applications.
Property | Value |
---|---|
Molecular Weight | 200.05 g/mol |
Density | 1.487 g/cm³ |
Boiling Point | 50 °C |
Refractive Index | 1.276 |
Cellular Effects
Research indicates that HFE-347pc-f significantly impacts cell function by modulating cell signaling pathways, gene expression, and cellular metabolism. Its ability to support stable cycling of NMC (Nickel Manganese Cobalt) and lithium metal phosphate cathodes is particularly noteworthy as it enhances ionic conduction while suppressing dendrite formation during battery operation.
Case Study: Impact on Lithium-Ion Batteries
A study highlighted the effectiveness of HFE-347pc-f in lithium-ion battery systems where it formed a protective interphase that improved cycle stability and efficiency. The compound's unique properties allowed for better performance under high-stress conditions, making it a candidate for next-generation battery technologies .
Applications in Research and Industry
HFE-347pc-f is employed in various scientific research applications due to its solvent properties and reactivity:
- As a Solvent: It serves as an environmentally friendly solvent in chemical processes.
- In Biological Assays: Its unique chemical structure makes it suitable for specific biological assays where traditional solvents may fail.
- Battery Technologies: Its role in enhancing battery performance has led to increased interest in its use within the energy sector .
Safety and Environmental Considerations
While HFE-347pc-f is classified as an irritant (Hazard Symbol: Xi), it is considered less harmful compared to other fluorinated compounds. It has been noted for its lower environmental impact when used as a cleaning agent or desiccant .
Table 2: Safety Data
Hazard Symbol | Risk Codes | Safety Description |
---|---|---|
Xi | 10 | Flammable |
Keep away from sources of ignition |
Q & A
Basic Research Questions
Q. What are the key physicochemical properties of 1,1,2,2-Tetrafluoroethyl 2,2,2-trifluoroethyl ether, and how do they influence its utility in experimental design?
Answer:
- Molecular Properties : Molecular formula C₄H₃F₇O, molecular weight 200.05 g/mol, boiling point 56–57°C (at 760 mmHg), density 1.479 g/cm³, and log Pow (octanol-water partition coefficient) 2.18 .
- Functional Utility : Low surface tension and viscosity enhance wetting and penetration in material compatibility studies. High fluorination enables dissolution of fluorinated polymers and inertness in reactive environments .
- Experimental Design Considerations : Select for reactions requiring non-polar, non-flammable solvents (flash point -6.96°C to 47°C, depending on purity) or low-GWP alternatives .
Q. How should researchers safely handle and store this compound in laboratory settings?
Answer:
- Hazards : Flammable (Category 2), skin/eye irritant (Category 2/2A) . Use PPE (gloves, goggles) and work in well-ventilated areas.
- Storage : Store in airtight containers at room temperature, away from ignition sources. Bulk storage in 25 kg/250 kg drums is recommended .
- Spill Management : Use non-sparking tools for cleanup; avoid discharge into waterways due to potential ecological effects (log Pow 2.18 suggests moderate bioaccumulation risk) .
Q. What analytical methods are recommended to validate the purity of this compound?
Answer:
- Gas Chromatography (GC) : Primary method for quantifying purity (≥98–99.9% as per commercial grades) .
- NMR Spectroscopy : Confirm structural integrity (e.g., absence of degradation products like trifluoroacetic acid).
- Density/Refractive Index : Cross-check against literature values (density 1.479 g/cm³; refractive index 1.276) .
Q. How can researchers optimize experimental protocols using this compound as a solvent for fluorinated compound synthesis?
Answer:
- Solvent Selection Criteria : Prioritize its low polarity (dielectric constant ~7.1) for dissolving fluorinated aromatics or perfluoropolymers .
- Reaction Compatibility : Ideal for low-temperature reactions (boiling point ~56°C) and electrochemical applications (e.g., lithium-sulfur batteries) .
- Post-Reaction Recovery : Distill at reduced pressure due to moderate volatility .
Advanced Research Questions
Q. How can the environmental impact of this compound be systematically assessed in long-term ecological studies?
Answer:
- Persistence Metrics : Atmospheric lifetime 5.99 years; GWP₁₀₀ = 964 (CO₂ = 1) .
- Bioaccumulation Potential : Log Pow 2.18 suggests moderate hydrophobicity, but insufficient data exist for definitive PBT (persistent, bioaccumulative, toxic) classification .
- Regulatory Alignment : Align with OECD 117 (log Pow), ISO 14507 (soil migration), and EPA guidelines for fluorinated ethers .
Q. What methodologies resolve discrepancies in reported purity and physicochemical data across commercial sources?
Answer:
- Comparative Analysis : Cross-validate purity via GC-MS and elemental analysis. For density discrepancies (1.434–1.479 g/cm³), standardize measurements at 20°C .
- Batch Variability : Trace impurities (e.g., residual methanol in some grades) may alter flammability or reactivity; use suppliers with ≥99.9% certification .
Q. What role does this compound play in advanced battery electrolytes, and how is its performance quantified?
Answer:
- Electrolyte Design : Acts as a co-solvent in lithium-sulfur batteries, improving ionic conductivity (e.g., 1.5–2.0 mS/cm at 25°C) and stabilizing lithium metal anodes .
- Performance Metrics : Electrochemical impedance spectroscopy (EIS) to assess interfacial stability; cyclic voltammetry for sulfur redox kinetics .
Q. How can researchers evaluate material compatibility with this compound in high-precision applications (e.g., microelectronics cleaning)?
Answer:
- Compatibility Testing : Immersion studies (24–72 hrs) on polymers (e.g., PTFE, PDMS) and metals (e.g., aluminum, copper) under controlled humidity/temperature .
- Surface Analysis : SEM/EDS to detect etching or swelling; contact angle measurements to assess wettability changes .
Properties
IUPAC Name |
1,1,2,2-tetrafluoro-1-(2,2,2-trifluoroethoxy)ethane | |
---|---|---|
Source | PubChem | |
URL | https://pubchem.ncbi.nlm.nih.gov | |
Description | Data deposited in or computed by PubChem | |
InChI |
InChI=1S/C4H3F7O/c5-2(6)4(10,11)12-1-3(7,8)9/h2H,1H2 | |
Source | PubChem | |
URL | https://pubchem.ncbi.nlm.nih.gov | |
Description | Data deposited in or computed by PubChem | |
InChI Key |
CWIFAKBLLXGZIC-UHFFFAOYSA-N | |
Source | PubChem | |
URL | https://pubchem.ncbi.nlm.nih.gov | |
Description | Data deposited in or computed by PubChem | |
Canonical SMILES |
C(C(F)(F)F)OC(C(F)F)(F)F | |
Source | PubChem | |
URL | https://pubchem.ncbi.nlm.nih.gov | |
Description | Data deposited in or computed by PubChem | |
Molecular Formula |
CF2HCF2OCH2CF3, C4H3F7O | |
Source | NORMAN Suspect List Exchange | |
Description | The NORMAN network enhances the exchange of information on emerging environmental substances, and encourages the validation and harmonisation of common measurement methods and monitoring tools so that the requirements of risk assessors and risk managers can be better met. The NORMAN Suspect List Exchange (NORMAN-SLE) is a central access point to find suspect lists relevant for various environmental monitoring questions, described in DOI:10.1186/s12302-022-00680-6 | |
Explanation | Data: CC-BY 4.0; Code (hosted by ECI, LCSB): Artistic-2.0 | |
Source | PubChem | |
URL | https://pubchem.ncbi.nlm.nih.gov | |
Description | Data deposited in or computed by PubChem | |
DSSTOX Substance ID |
DTXSID8074786 | |
Record name | (1H,1H-Perfluoroethyl)(2H-perfluoroethyl)ether | |
Source | EPA DSSTox | |
URL | https://comptox.epa.gov/dashboard/DTXSID8074786 | |
Description | DSSTox provides a high quality public chemistry resource for supporting improved predictive toxicology. | |
Molecular Weight |
200.05 g/mol | |
Source | PubChem | |
URL | https://pubchem.ncbi.nlm.nih.gov | |
Description | Data deposited in or computed by PubChem | |
Physical Description |
Liquid | |
Record name | Ethane, 1,1,2,2-tetrafluoro-1-(2,2,2-trifluoroethoxy)- | |
Source | EPA Chemicals under the TSCA | |
URL | https://www.epa.gov/chemicals-under-tsca | |
Description | EPA Chemicals under the Toxic Substances Control Act (TSCA) collection contains information on chemicals and their regulations under TSCA, including non-confidential content from the TSCA Chemical Substance Inventory and Chemical Data Reporting. | |
CAS No. |
406-78-0 | |
Record name | 1,1,2,2-Tetrafluoro-1-(2,2,2-trifluoroethoxy)ethane | |
Source | CAS Common Chemistry | |
URL | https://commonchemistry.cas.org/detail?cas_rn=406-78-0 | |
Description | CAS Common Chemistry is an open community resource for accessing chemical information. Nearly 500,000 chemical substances from CAS REGISTRY cover areas of community interest, including common and frequently regulated chemicals, and those relevant to high school and undergraduate chemistry classes. This chemical information, curated by our expert scientists, is provided in alignment with our mission as a division of the American Chemical Society. | |
Explanation | The data from CAS Common Chemistry is provided under a CC-BY-NC 4.0 license, unless otherwise stated. | |
Record name | 1,1,2,2-Tetrafluoro-1-(2,2,2-trifluoroethoxy)ethane | |
Source | ChemIDplus | |
URL | https://pubchem.ncbi.nlm.nih.gov/substance/?source=chemidplus&sourceid=0000406780 | |
Description | ChemIDplus is a free, web search system that provides access to the structure and nomenclature authority files used for the identification of chemical substances cited in National Library of Medicine (NLM) databases, including the TOXNET system. | |
Record name | Ethane, 1,1,2,2-tetrafluoro-1-(2,2,2-trifluoroethoxy)- | |
Source | EPA Chemicals under the TSCA | |
URL | https://www.epa.gov/chemicals-under-tsca | |
Description | EPA Chemicals under the Toxic Substances Control Act (TSCA) collection contains information on chemicals and their regulations under TSCA, including non-confidential content from the TSCA Chemical Substance Inventory and Chemical Data Reporting. | |
Record name | (1H,1H-Perfluoroethyl)(2H-perfluoroethyl)ether | |
Source | EPA DSSTox | |
URL | https://comptox.epa.gov/dashboard/DTXSID8074786 | |
Description | DSSTox provides a high quality public chemistry resource for supporting improved predictive toxicology. | |
Retrosynthesis Analysis
AI-Powered Synthesis Planning: Our tool employs the Template_relevance Pistachio, Template_relevance Bkms_metabolic, Template_relevance Pistachio_ringbreaker, Template_relevance Reaxys, Template_relevance Reaxys_biocatalysis model, leveraging a vast database of chemical reactions to predict feasible synthetic routes.
One-Step Synthesis Focus: Specifically designed for one-step synthesis, it provides concise and direct routes for your target compounds, streamlining the synthesis process.
Accurate Predictions: Utilizing the extensive PISTACHIO, BKMS_METABOLIC, PISTACHIO_RINGBREAKER, REAXYS, REAXYS_BIOCATALYSIS database, our tool offers high-accuracy predictions, reflecting the latest in chemical research and data.
Strategy Settings
Precursor scoring | Relevance Heuristic |
---|---|
Min. plausibility | 0.01 |
Model | Template_relevance |
Template Set | Pistachio/Bkms_metabolic/Pistachio_ringbreaker/Reaxys/Reaxys_biocatalysis |
Top-N result to add to graph | 6 |
Feasible Synthetic Routes
Disclaimer and Information on In-Vitro Research Products
Please be aware that all articles and product information presented on BenchChem are intended solely for informational purposes. The products available for purchase on BenchChem are specifically designed for in-vitro studies, which are conducted outside of living organisms. In-vitro studies, derived from the Latin term "in glass," involve experiments performed in controlled laboratory settings using cells or tissues. It is important to note that these products are not categorized as medicines or drugs, and they have not received approval from the FDA for the prevention, treatment, or cure of any medical condition, ailment, or disease. We must emphasize that any form of bodily introduction of these products into humans or animals is strictly prohibited by law. It is essential to adhere to these guidelines to ensure compliance with legal and ethical standards in research and experimentation.