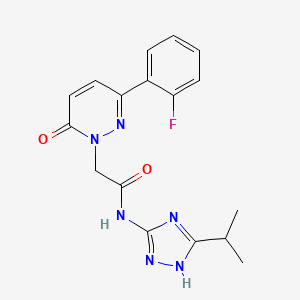
C17H17FN6O2
- Click on QUICK INQUIRY to receive a quote from our team of experts.
- With the quality product at a COMPETITIVE price, you can focus more on your research.
Overview
Description
Preparation Methods
Synthetic Routes and Reaction Conditions
The synthesis of 2-(2-aminoethoxy)-5-fluoro-N-{[3-(2H-1,2,3,4-tetrazol-5-yl)phenyl]methyl}benzamide involves several steps. One common method includes the reaction of 5-fluoro-2-nitrobenzoic acid with 2-aminoethanol to form an intermediate, which is then reduced to the corresponding amine. This amine is further reacted with 3-(2H-1,2,3,4-tetrazol-5-yl)benzyl chloride under specific conditions to yield the final product .
Industrial Production Methods
In industrial settings, the production of this compound may involve large-scale synthesis using optimized reaction conditions to ensure high yield and purity. Techniques such as solid-phase synthesis , gas-phase synthesis , and liquid-phase synthesis are commonly employed . These methods are designed to be efficient and environmentally friendly, minimizing waste and energy consumption.
Chemical Reactions Analysis
Types of Reactions
2-(2-aminoethoxy)-5-fluoro-N-{[3-(2H-1,2,3,4-tetrazol-5-yl)phenyl]methyl}benzamide: undergoes various chemical reactions, including:
Oxidation: This compound can be oxidized using reagents like or .
Reduction: Reduction reactions can be carried out using or .
Substitution: Nucleophilic substitution reactions can occur with reagents such as sodium azide or sodium methoxide .
Common Reagents and Conditions
The reactions typically require specific conditions, such as controlled temperature, pH, and solvent choice. For example, oxidation reactions may be performed in an acidic medium, while reduction reactions often require anhydrous conditions .
Major Products Formed
The major products formed from these reactions depend on the specific reagents and conditions used. For instance, oxidation may yield carboxylic acids , while reduction can produce amines or alcohols .
Scientific Research Applications
2-(2-aminoethoxy)-5-fluoro-N-{[3-(2H-1,2,3,4-tetrazol-5-yl)phenyl]methyl}benzamide: has a wide range of applications in scientific research:
Mechanism of Action
The mechanism of action of 2-(2-aminoethoxy)-5-fluoro-N-{[3-(2H-1,2,3,4-tetrazol-5-yl)phenyl]methyl}benzamide involves its interaction with specific molecular targets. It can bind to enzymes or receptors, modulating their activity and triggering downstream signaling pathways . The exact molecular targets and pathways depend on the specific application and context in which the compound is used .
Comparison with Similar Compounds
Similar Compounds
Some compounds similar to 2-(2-aminoethoxy)-5-fluoro-N-{[3-(2H-1,2,3,4-tetrazol-5-yl)phenyl]methyl}benzamide include:
- 2-(2-aminoethoxy)-5-fluoro-N-{[3-(2H-1,2,3,4-tetrazol-5-yl)phenyl]methyl}benzamide
- 8-[(2Z)-2-[(4-fluorophenyl)methylidene]hydrazinyl]-1,3-dimethyl-7-prop-2-enylpurine-2,6-dione
Uniqueness
The uniqueness of 2-(2-aminoethoxy)-5-fluoro-N-{[3-(2H-1,2,3,4-tetrazol-5-yl)phenyl]methyl}benzamide lies in its specific chemical structure, which imparts distinct properties and reactivity. This makes it valuable for specialized applications in research and industry .
Biological Activity
C17H17FN6O2 is a fluorinated derivative of 2-deoxy-D-glucose (2-DG), a compound that has garnered attention for its potential therapeutic applications, particularly in cancer treatment. This article explores the biological activity of this compound, focusing on its mechanisms of action, cytotoxicity, and implications for cancer therapy.
Background
2-DG is a glucose analog that inhibits glycolysis, making it a candidate for cancer therapies, especially in tumors with high glycolytic rates like glioblastoma multiforme (GBM). However, its clinical use has been limited due to poor pharmacokinetics. Modifications to 2-DG, such as the introduction of fluorine atoms, have been investigated to enhance its biological activity and therapeutic efficacy.
The primary mechanism by which this compound exerts its effects is through the inhibition of hexokinase (HK), an enzyme crucial for the glycolytic pathway. Upon cellular uptake, 2-DG is phosphorylated to 2-DG-6-phosphate, which competes with glucose and inhibits HK activity. The fluorinated derivatives have shown enhanced binding affinity to HK compared to 2-DG, leading to improved inhibition of glycolysis.
Key Findings from Research
- Cytotoxicity : Fluorinated derivatives, including this compound, exhibit potent cytotoxic effects against cancer cells. Studies indicate that these compounds have lower IC50 values than 2-DG, particularly under hypoxic conditions typical of tumor microenvironments .
- Hexokinase Inhibition : Enzymatic assays demonstrated that this compound and other halogenated derivatives are more effective inhibitors of HKII than 2-DG. This enhanced inhibition is attributed to the structural modifications that improve stability and cellular uptake .
- Molecular Interactions : NMR spectroscopy and molecular docking studies reveal that fluorinated compounds bind to HK similarly to glucose, suggesting a competitive inhibition mechanism. This binding affinity translates into increased efficacy in reducing glycolytic flux in cancer cells .
Case Studies
Several case studies have illustrated the potential of this compound in clinical settings:
- Glioblastoma Treatment : In vitro studies on GBM cell lines showed that treatment with this compound resulted in significant reductions in cell viability compared to untreated controls. The compound's ability to induce apoptosis was confirmed through flow cytometry analysis .
- Combination Therapies : Research indicates that combining this compound with other chemotherapeutic agents may enhance overall treatment efficacy. For instance, synergistic effects were observed when used alongside standard GBM therapies, suggesting a multi-faceted approach could improve patient outcomes .
Data Table: Biological Activity Summary
Parameter | This compound | 2-Deoxy-D-Glucose (2-DG) |
---|---|---|
IC50 (Cancer Cells) | Lower than 10 µM | Approximately 30 µM |
Hexokinase Inhibition | Stronger | Moderate |
Apoptosis Induction | Yes | Limited |
Stability in Biological Systems | Enhanced | Poor |
Properties
Molecular Formula |
C17H17FN6O2 |
---|---|
Molecular Weight |
356.4 g/mol |
IUPAC Name |
2-[3-(2-fluorophenyl)-6-oxopyridazin-1-yl]-N-(5-propan-2-yl-1H-1,2,4-triazol-3-yl)acetamide |
InChI |
InChI=1S/C17H17FN6O2/c1-10(2)16-20-17(22-21-16)19-14(25)9-24-15(26)8-7-13(23-24)11-5-3-4-6-12(11)18/h3-8,10H,9H2,1-2H3,(H2,19,20,21,22,25) |
InChI Key |
MRGIRFVDPSONKP-UHFFFAOYSA-N |
Canonical SMILES |
CC(C)C1=NC(=NN1)NC(=O)CN2C(=O)C=CC(=N2)C3=CC=CC=C3F |
Origin of Product |
United States |
Disclaimer and Information on In-Vitro Research Products
Please be aware that all articles and product information presented on BenchChem are intended solely for informational purposes. The products available for purchase on BenchChem are specifically designed for in-vitro studies, which are conducted outside of living organisms. In-vitro studies, derived from the Latin term "in glass," involve experiments performed in controlled laboratory settings using cells or tissues. It is important to note that these products are not categorized as medicines or drugs, and they have not received approval from the FDA for the prevention, treatment, or cure of any medical condition, ailment, or disease. We must emphasize that any form of bodily introduction of these products into humans or animals is strictly prohibited by law. It is essential to adhere to these guidelines to ensure compliance with legal and ethical standards in research and experimentation.