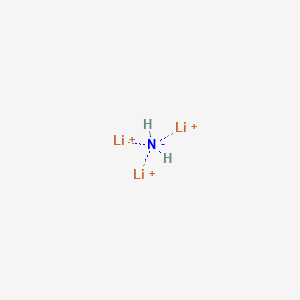
Lithium nitride
Overview
Description
It is the only stable alkali metal nitride and appears as a reddish-pink solid with a high melting point . This compound is notable for its unique properties and applications in various scientific fields.
Mechanism of Action
Target of Action
Lithium nitride (Li3N) primarily targets nitrogen molecules (N2) . It is capable of breaking the triple-bonded nitrogen molecule under ambient conditions . In the context of lithium metal batteries, Li3N is often found in the solid-electrolyte interphases (SEIs) on lithium metal anodes .
Mode of Action
Li3N interacts with its targets through a series of chemical reactions. For instance, it is prepared by the direct reaction of elemental lithium with nitrogen gas . The reaction is as follows:
6Li+N2→2Li3N6Li + N2 \rightarrow 2Li3N 6Li+N2→2Li3N
In the context of lithium metal batteries, Li3N guides lithium electrodeposition along its surface, decreases Li±solvent coordination, induces organic-poor SEI on the lithium metal anode, and facilitates Li+ transport in the electrolyte .
Biochemical Pathways
The biochemical pathways involving Li3N are primarily related to the synthesis of ammonia. Ammonia synthesis chemistry with lithium–nitrogen–hydrogen materials is largely confined to pathways involving lithium hydride and lithium imide . An alternate pathway featuring this compound–hydride shows more favorable characteristics from an activity, synthesis, and cyclability perspective .
Result of Action
The result of Li3N’s action is the formation of a compound with unique properties. For instance, in the presence of nitrogen gas, Li3N is formed . When Li3N comes into contact with water, it reacts violently to produce ammonia :
Li3N+3H2O→3LiOH+NH3Li3N + 3H2O \rightarrow 3LiOH + NH3 Li3N+3H2O→3LiOH+NH3
In the context of lithium metal batteries, the presence of Li3N in the SEIs improves the electrochemical performance of the batteries .
Action Environment
The action of Li3N is influenced by environmental factors. For instance, Li3N must be protected from moisture as it reacts violently with water to produce ammonia . In the context of lithium metal batteries, the electrochemical performance of Li3N is affected by both the SEIs and the electrolyte species . Furthermore, the extraction of lithium from salt lakes has been revolutionized by the development of a crystalline carbon nitride membrane, which shows remarkable efficiency and durability in separating lithium ions from magnesium ions in salt-lake brine .
Preparation Methods
Lithium nitride can be synthesized through the direct reaction of elemental lithium with nitrogen gas: [ 6 \text{Li} + \text{N}_2 \rightarrow 2 \text{Li}_3\text{N} ] Alternatively, a solution of lithium in liquid sodium metal can be treated with nitrogen gas to produce trithis compound . Industrial production methods typically involve placing lithium metal under a nitrogen environment .
Chemical Reactions Analysis
Lithium nitride undergoes several types of chemical reactions:
Reaction with Water: It reacts violently with water to produce lithium hydroxide and ammonia[ \text{Li}_3\text{N} + 3 \text{H}_2\text{O} \rightarrow 3 \text{LiOH} + \text{NH}_3 ]
Reaction with Carbon Dioxide: It reacts with carbon dioxide to form amorphous carbon nitride and lithium cyanamide[ \text{Li}_3\text{N} + 3 \text{CO}_2 \rightarrow \text{C}_3\text{N}_4 + 3 \text{Li}_2\text{CN}_2 ]
Reaction with Hydrogen: Under hydrogen at around 200°C, trithis compound forms lithium amide[ \text{Li}_3\text{N} + \text{H}_2 \rightarrow \text{LiNH}_2 ]
Scientific Research Applications
Lithium nitride has a wide range of applications in scientific research:
Hydrogen Storage: It is used as a hydrogen storage material due to its ability to absorb and release hydrogen efficiently.
Organic Light-Emitting Diodes (OLEDs): It serves as an electron injection material in OLEDs.
Lithium-Ion Batteries: This compound is utilized in the fabrication of lithium-ion batteries, contributing to their high energy density and efficiency.
Solid-State Ionic Conductor: It is known for its high ionic conductivity and is used in various solid-state devices.
Comparison with Similar Compounds
Lithium nitride can be compared with other similar compounds, such as:
- Lithium Phosphide (Li₃P)
- Lithium Arsenide (Li₃As)
- Lithium Fluoride (LiF)
- Lithium Oxide (Li₂O)
- Tetralithiomethane (Li₄C)
- Lithium Carbide (Li₂C₂)
What sets trithis compound apart is its unique stability as the only stable alkali metal nitride and its high ionic conductivity .
Properties
IUPAC Name |
trilithium;azanide | |
---|---|---|
Source | PubChem | |
URL | https://pubchem.ncbi.nlm.nih.gov | |
Description | Data deposited in or computed by PubChem | |
InChI |
InChI=1S/3Li.H2N/h;;;1H2/q3*+1;-1 | |
Source | PubChem | |
URL | https://pubchem.ncbi.nlm.nih.gov | |
Description | Data deposited in or computed by PubChem | |
InChI Key |
BHZCMUVGYXEBMY-UHFFFAOYSA-N | |
Source | PubChem | |
URL | https://pubchem.ncbi.nlm.nih.gov | |
Description | Data deposited in or computed by PubChem | |
Canonical SMILES |
[Li+].[Li+].[Li+].[NH2-] | |
Source | PubChem | |
URL | https://pubchem.ncbi.nlm.nih.gov | |
Description | Data deposited in or computed by PubChem | |
Molecular Formula |
H2Li3N+2 | |
Source | PubChem | |
URL | https://pubchem.ncbi.nlm.nih.gov | |
Description | Data deposited in or computed by PubChem | |
DSSTOX Substance ID |
DTXSID80894085 | |
Record name | Lithium nitride | |
Source | EPA DSSTox | |
URL | https://comptox.epa.gov/dashboard/DTXSID80894085 | |
Description | DSSTox provides a high quality public chemistry resource for supporting improved predictive toxicology. | |
Molecular Weight |
36.9 g/mol | |
Source | PubChem | |
URL | https://pubchem.ncbi.nlm.nih.gov | |
Description | Data deposited in or computed by PubChem | |
Physical Description |
Lithium nitride appears as a reddish brown powder. Insoluble in most organic solvents. Used in metallurgy and chemical synthesis., Brownish-red solid or powder; [NJ-HSFS] | |
Record name | LITHIUM NITRIDE | |
Source | CAMEO Chemicals | |
URL | https://cameochemicals.noaa.gov/chemical/1001 | |
Description | CAMEO Chemicals is a chemical database designed for people who are involved in hazardous material incident response and planning. CAMEO Chemicals contains a library with thousands of datasheets containing response-related information and recommendations for hazardous materials that are commonly transported, used, or stored in the United States. CAMEO Chemicals was developed by the National Oceanic and Atmospheric Administration's Office of Response and Restoration in partnership with the Environmental Protection Agency's Office of Emergency Management. | |
Explanation | CAMEO Chemicals and all other CAMEO products are available at no charge to those organizations and individuals (recipients) responsible for the safe handling of chemicals. However, some of the chemical data itself is subject to the copyright restrictions of the companies or organizations that provided the data. | |
Record name | Lithium nitride | |
Source | Haz-Map, Information on Hazardous Chemicals and Occupational Diseases | |
URL | https://haz-map.com/Agents/1619 | |
Description | Haz-Map® is an occupational health database designed for health and safety professionals and for consumers seeking information about the adverse effects of workplace exposures to chemical and biological agents. | |
Explanation | Copyright (c) 2022 Haz-Map(R). All rights reserved. Unless otherwise indicated, all materials from Haz-Map are copyrighted by Haz-Map(R). No part of these materials, either text or image may be used for any purpose other than for personal use. Therefore, reproduction, modification, storage in a retrieval system or retransmission, in any form or by any means, electronic, mechanical or otherwise, for reasons other than personal use, is strictly prohibited without prior written permission. | |
CAS No. |
26134-62-3 | |
Record name | LITHIUM NITRIDE | |
Source | CAMEO Chemicals | |
URL | https://cameochemicals.noaa.gov/chemical/1001 | |
Description | CAMEO Chemicals is a chemical database designed for people who are involved in hazardous material incident response and planning. CAMEO Chemicals contains a library with thousands of datasheets containing response-related information and recommendations for hazardous materials that are commonly transported, used, or stored in the United States. CAMEO Chemicals was developed by the National Oceanic and Atmospheric Administration's Office of Response and Restoration in partnership with the Environmental Protection Agency's Office of Emergency Management. | |
Explanation | CAMEO Chemicals and all other CAMEO products are available at no charge to those organizations and individuals (recipients) responsible for the safe handling of chemicals. However, some of the chemical data itself is subject to the copyright restrictions of the companies or organizations that provided the data. | |
Record name | Lithium nitride (Li3N) | |
Source | ChemIDplus | |
URL | https://pubchem.ncbi.nlm.nih.gov/substance/?source=chemidplus&sourceid=0026134623 | |
Description | ChemIDplus is a free, web search system that provides access to the structure and nomenclature authority files used for the identification of chemical substances cited in National Library of Medicine (NLM) databases, including the TOXNET system. | |
Record name | Lithium nitride (Li3N) | |
Source | EPA Chemicals under the TSCA | |
URL | https://www.epa.gov/chemicals-under-tsca | |
Description | EPA Chemicals under the Toxic Substances Control Act (TSCA) collection contains information on chemicals and their regulations under TSCA, including non-confidential content from the TSCA Chemical Substance Inventory and Chemical Data Reporting. | |
Record name | Lithium nitride | |
Source | EPA DSSTox | |
URL | https://comptox.epa.gov/dashboard/DTXSID80894085 | |
Description | DSSTox provides a high quality public chemistry resource for supporting improved predictive toxicology. | |
Record name | Trilithium nitride | |
Source | European Chemicals Agency (ECHA) | |
URL | https://echa.europa.eu/substance-information/-/substanceinfo/100.043.144 | |
Description | The European Chemicals Agency (ECHA) is an agency of the European Union which is the driving force among regulatory authorities in implementing the EU's groundbreaking chemicals legislation for the benefit of human health and the environment as well as for innovation and competitiveness. | |
Explanation | Use of the information, documents and data from the ECHA website is subject to the terms and conditions of this Legal Notice, and subject to other binding limitations provided for under applicable law, the information, documents and data made available on the ECHA website may be reproduced, distributed and/or used, totally or in part, for non-commercial purposes provided that ECHA is acknowledged as the source: "Source: European Chemicals Agency, http://echa.europa.eu/". Such acknowledgement must be included in each copy of the material. ECHA permits and encourages organisations and individuals to create links to the ECHA website under the following cumulative conditions: Links can only be made to webpages that provide a link to the Legal Notice page. | |
Disclaimer and Information on In-Vitro Research Products
Please be aware that all articles and product information presented on BenchChem are intended solely for informational purposes. The products available for purchase on BenchChem are specifically designed for in-vitro studies, which are conducted outside of living organisms. In-vitro studies, derived from the Latin term "in glass," involve experiments performed in controlled laboratory settings using cells or tissues. It is important to note that these products are not categorized as medicines or drugs, and they have not received approval from the FDA for the prevention, treatment, or cure of any medical condition, ailment, or disease. We must emphasize that any form of bodily introduction of these products into humans or animals is strictly prohibited by law. It is essential to adhere to these guidelines to ensure compliance with legal and ethical standards in research and experimentation.