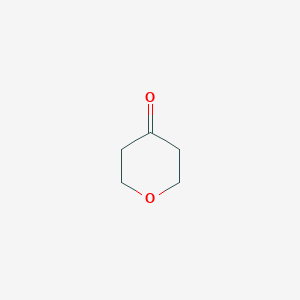
Tetrahydro-4H-pyran-4-one
Overview
Description
Tetrahydro-4H-pyran-4-one is a useful research compound. Its molecular formula is C5H8O2 and its molecular weight is 100.12 g/mol. The purity is usually 95%.
The exact mass of the compound this compound is unknown and the complexity rating of the compound is unknown. The United Nations designated GHS hazard class pictogram is Flammable;Irritant, and the GHS signal word is WarningThe storage condition is unknown. Please store according to label instructions upon receipt of goods.
BenchChem offers high-quality this compound suitable for many research applications. Different packaging options are available to accommodate customers' requirements. Please inquire for more information about this compound including the price, delivery time, and more detailed information at info@benchchem.com.
Mechanism of Action
Target of Action
Tetrahydro-4H-pyran-4-one has shown potential applications in the modulation of NMDA receptors . NMDA receptors play a crucial role in excitatory synaptic transmission and are involved in various brain functions, such as learning and memory .
Mode of Action
The oxygen atom in the pyran ring of this compound acts as a nucleophile , readily participating in reactions such as acylation, alkylation, and condensation reactions . This makes it useful for functionalization and synthesis of organic compounds .
Result of Action
The molecular and cellular effects of this compound’s action are likely tied to its modulation of NMDA receptors . By influencing these receptors, it could potentially affect neuronal signaling and synaptic transmission, impacting brain functions like learning and memory .
Action Environment
This compound is stable under normal conditions, but can degrade when exposed to heat, light, or oxidizing agents . Its water solubility suggests it may be mobile in the environment . These factors could influence the compound’s action, efficacy, and stability.
Biochemical Analysis
Biochemical Properties
Tetrahydro-4H-pyran-4-one exhibits reactivity towards nucleophiles and electrophiles, making it useful for functionalization and synthesis of organic compounds . The oxygen atom in the pyran ring acts as a nucleophile, readily participating in reactions such as acylation, alkylation, and condensation reactions .
Cellular Effects
This compound has potential biological activities, including antimicrobial and insecticidal properties . Additionally, this compound and a known compound derived from it have shown anti-influenza virus activity, indicating their potential as candidates for antiviral drug development .
Molecular Mechanism
Its unique reactivity towards nucleophiles and electrophiles suggests that it may interact with various biomolecules in a cell, potentially influencing enzyme activity and gene expression .
Temporal Effects in Laboratory Settings
This compound is stable under normal conditions, but can degrade when exposed to heat, light, or oxidizing agents
Dosage Effects in Animal Models
The effects of this compound vary with different dosages in animal models
Metabolic Pathways
It is likely that it interacts with various enzymes or cofactors, and could potentially affect metabolic flux or metabolite levels .
Transport and Distribution
It is likely that it interacts with various transporters or binding proteins, and could potentially affect its localization or accumulation .
Subcellular Localization
It could potentially be directed to specific compartments or organelles by targeting signals or post-translational modifications .
Properties
IUPAC Name |
oxan-4-one | |
---|---|---|
Source | PubChem | |
URL | https://pubchem.ncbi.nlm.nih.gov | |
Description | Data deposited in or computed by PubChem | |
InChI |
InChI=1S/C5H8O2/c6-5-1-3-7-4-2-5/h1-4H2 | |
Source | PubChem | |
URL | https://pubchem.ncbi.nlm.nih.gov | |
Description | Data deposited in or computed by PubChem | |
InChI Key |
JMJRYTGVHCAYCT-UHFFFAOYSA-N | |
Source | PubChem | |
URL | https://pubchem.ncbi.nlm.nih.gov | |
Description | Data deposited in or computed by PubChem | |
Canonical SMILES |
C1COCCC1=O | |
Source | PubChem | |
URL | https://pubchem.ncbi.nlm.nih.gov | |
Description | Data deposited in or computed by PubChem | |
Molecular Formula |
C5H8O2 | |
Source | PubChem | |
URL | https://pubchem.ncbi.nlm.nih.gov | |
Description | Data deposited in or computed by PubChem | |
DSSTOX Substance ID |
DTXSID70184048 | |
Record name | Tetrahydro-4H-pyran-4-one | |
Source | EPA DSSTox | |
URL | https://comptox.epa.gov/dashboard/DTXSID70184048 | |
Description | DSSTox provides a high quality public chemistry resource for supporting improved predictive toxicology. | |
Molecular Weight |
100.12 g/mol | |
Source | PubChem | |
URL | https://pubchem.ncbi.nlm.nih.gov | |
Description | Data deposited in or computed by PubChem | |
CAS No. |
29943-42-8 | |
Record name | Tetrahydro-4H-pyran-4-one | |
Source | CAS Common Chemistry | |
URL | https://commonchemistry.cas.org/detail?cas_rn=29943-42-8 | |
Description | CAS Common Chemistry is an open community resource for accessing chemical information. Nearly 500,000 chemical substances from CAS REGISTRY cover areas of community interest, including common and frequently regulated chemicals, and those relevant to high school and undergraduate chemistry classes. This chemical information, curated by our expert scientists, is provided in alignment with our mission as a division of the American Chemical Society. | |
Explanation | The data from CAS Common Chemistry is provided under a CC-BY-NC 4.0 license, unless otherwise stated. | |
Record name | Tetrahydro-4H-pyran-4-one | |
Source | ChemIDplus | |
URL | https://pubchem.ncbi.nlm.nih.gov/substance/?source=chemidplus&sourceid=0029943428 | |
Description | ChemIDplus is a free, web search system that provides access to the structure and nomenclature authority files used for the identification of chemical substances cited in National Library of Medicine (NLM) databases, including the TOXNET system. | |
Record name | Tetrahydro-4H-pyran-4-one | |
Source | EPA DSSTox | |
URL | https://comptox.epa.gov/dashboard/DTXSID70184048 | |
Description | DSSTox provides a high quality public chemistry resource for supporting improved predictive toxicology. | |
Record name | Tetrahydro-4H-pyran-4-one | |
Source | European Chemicals Agency (ECHA) | |
URL | https://echa.europa.eu/substance-information/-/substanceinfo/100.045.410 | |
Description | The European Chemicals Agency (ECHA) is an agency of the European Union which is the driving force among regulatory authorities in implementing the EU's groundbreaking chemicals legislation for the benefit of human health and the environment as well as for innovation and competitiveness. | |
Explanation | Use of the information, documents and data from the ECHA website is subject to the terms and conditions of this Legal Notice, and subject to other binding limitations provided for under applicable law, the information, documents and data made available on the ECHA website may be reproduced, distributed and/or used, totally or in part, for non-commercial purposes provided that ECHA is acknowledged as the source: "Source: European Chemicals Agency, http://echa.europa.eu/". Such acknowledgement must be included in each copy of the material. ECHA permits and encourages organisations and individuals to create links to the ECHA website under the following cumulative conditions: Links can only be made to webpages that provide a link to the Legal Notice page. | |
Retrosynthesis Analysis
AI-Powered Synthesis Planning: Our tool employs the Template_relevance Pistachio, Template_relevance Bkms_metabolic, Template_relevance Pistachio_ringbreaker, Template_relevance Reaxys, Template_relevance Reaxys_biocatalysis model, leveraging a vast database of chemical reactions to predict feasible synthetic routes.
One-Step Synthesis Focus: Specifically designed for one-step synthesis, it provides concise and direct routes for your target compounds, streamlining the synthesis process.
Accurate Predictions: Utilizing the extensive PISTACHIO, BKMS_METABOLIC, PISTACHIO_RINGBREAKER, REAXYS, REAXYS_BIOCATALYSIS database, our tool offers high-accuracy predictions, reflecting the latest in chemical research and data.
Strategy Settings
Precursor scoring | Relevance Heuristic |
---|---|
Min. plausibility | 0.01 |
Model | Template_relevance |
Template Set | Pistachio/Bkms_metabolic/Pistachio_ringbreaker/Reaxys/Reaxys_biocatalysis |
Top-N result to add to graph | 6 |
Feasible Synthetic Routes
Q1: What is the molecular formula and weight of Tetrahydro-4H-pyran-4-one?
A1: this compound has the molecular formula C5H8O2 and a molecular weight of 100.12 g/mol.
Q2: What spectroscopic data is available for characterizing this compound?
A2: Researchers commonly utilize IR, 1H NMR, and 13C NMR spectroscopy to characterize this compound. [, , ] Additionally, far-infrared spectroscopy has been employed to study its conformational dynamics, particularly chair inversion. []
Q3: How can this compound be synthesized?
A3: Several synthetic routes exist for this compound. One efficient two-step method starts with 3-chloropropionyl chloride, achieving a 45% overall yield. [] Another approach involves a Lewis acid-catalyzed [4+2] cycloaddition of 3-alkoxycyclobutanones with aldehydes or ketones. [] Additionally, 1,1-diethoxybut-2-ene serves as a precursor for synthesizing this compound derivatives. [, ]
Q4: What types of reactions can this compound undergo?
A4: this compound is a valuable starting material for synthesizing various heterocyclic compounds. [] For instance, it readily undergoes aldol condensation with aromatic aldehydes, leading to 3,5-dibenzylidene-tetrahydropyran-4-one derivatives. [] It also reacts with aryl Grignard reagents to form tertiary alcohols. [] Furthermore, its derivatives participate in reactions with sulfene and dichloroketene, yielding new heterocyclic systems like 5H-pyrano[3,4-e]-1,2-oxathiin and 2H,5H-Pyrano[4,3-b]pyran derivatives. []
Q5: What are some applications of this compound derivatives?
A6: this compound derivatives have found applications in medicinal chemistry and materials science. For instance, they serve as intermediates in the synthesis of TAK-779, a non-peptide CCR5 antagonist. [, ] Additionally, they act as precursors to 4-substituted quinuclidines and 1-phosphabicyclo[2.2.2]octanes. []
Q6: Have any biologically active this compound derivatives been reported?
A7: Yes, researchers have synthesized and evaluated the biological activity of several this compound derivatives. For instance, tetrahydro-4-aryl-3-[(dimethylamino)methyl]-2H-pyranols, derived from this compound, displayed analgesic activity comparable to codeine. [] Furthermore, in silico studies on 3,5-bis(hydroxymethyl)this compound derivatives predicted potential antidiabetic activity and activity in treating phobic disorders and dementia. []
Q7: Has this compound been used in the synthesis of porphyrins?
A8: Yes, this compound has been successfully incorporated into porphyrin structures, specifically for synthesizing porphyrins with a fused cyclic ether subunit. []
Q8: Have computational methods been applied to study this compound and its derivatives?
A9: Yes, computational studies have provided valuable insights into the properties of this compound and its derivatives. For example, quantum-chemical calculations using the DFT B3LYP method with 6-31G(d) and 6-311+G(3df,2p) basis sets were employed to determine the stable conformations of 3,5-bis(hydroxymethyl)this compound. [] Furthermore, in silico drug-likeness, biological activity, and toxicity predictions have been performed on novel derivatives. []
Q9: What is known about the Structure-Activity Relationship (SAR) of this compound derivatives?
A10: While the provided research doesn't explicitly detail specific SAR trends for this compound, it's clear that modifications to the core structure significantly influence its reactivity and biological activity. For instance, introducing aryl substituents at the 4-position impacts its behavior in reactions with Grignard reagents. [] Additionally, the nature of the N-substituents in N,N-disubstituted (E)-3-aminomethylene-2,3,5,6-tetrahydro-4H-pyran-4-ones dictates their reactivity with sulfene and dichloroketene. []
Disclaimer and Information on In-Vitro Research Products
Please be aware that all articles and product information presented on BenchChem are intended solely for informational purposes. The products available for purchase on BenchChem are specifically designed for in-vitro studies, which are conducted outside of living organisms. In-vitro studies, derived from the Latin term "in glass," involve experiments performed in controlled laboratory settings using cells or tissues. It is important to note that these products are not categorized as medicines or drugs, and they have not received approval from the FDA for the prevention, treatment, or cure of any medical condition, ailment, or disease. We must emphasize that any form of bodily introduction of these products into humans or animals is strictly prohibited by law. It is essential to adhere to these guidelines to ensure compliance with legal and ethical standards in research and experimentation.