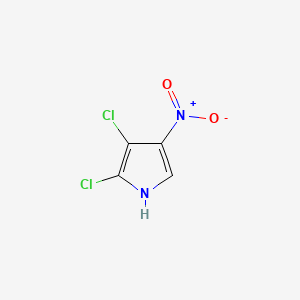
Pyrrolomycin A
Overview
Description
Pyrrolomycin A is a member of the pyrrolomycin family, which are natural antibiotics produced by various species of the Streptomyces genus. These compounds are known for their potent antibacterial activity, particularly against Gram-positive bacteria. This compound is characterized by its heavily halogenated structure, which contributes to its biological activity .
Preparation Methods
Synthetic Routes and Reaction Conditions
Pyrrolomycin A can be synthesized through a series of chemical reactions involving halogenation and cyclization processesThe reaction conditions often involve the use of halogenating agents such as chlorine or bromine, and the reactions are carried out under controlled temperatures and pressures to ensure the desired product is obtained .
Industrial Production Methods
Industrial production of this compound involves the fermentation of Streptomyces species that naturally produce this compound. The fermentation process is optimized to maximize the yield of this compound, and the compound is subsequently extracted and purified using various chromatographic techniques .
Chemical Reactions Analysis
Types of Reactions
Pyrrolomycin A undergoes several types of chemical reactions, including:
Oxidation: this compound can be oxidized to form various oxidized derivatives.
Reduction: Reduction reactions can be used to modify the halogenated groups on the pyrrole ring.
Substitution: Halogen atoms on this compound can be substituted with other functional groups to create new derivatives.
Common Reagents and Conditions
Common reagents used in the reactions of this compound include halogenating agents (e.g., chlorine, bromine), oxidizing agents (e.g., potassium permanganate), and reducing agents (e.g., sodium borohydride). The reactions are typically carried out under controlled conditions to ensure the desired products are formed .
Major Products Formed
The major products formed from the reactions of this compound include various halogenated derivatives, oxidized compounds, and substituted pyrrole derivatives.
Scientific Research Applications
Pyrrolomycin A has a wide range of scientific research applications, including:
Chemistry: this compound is used as a model compound for studying halogenation reactions and the synthesis of halogenated pyrrole derivatives.
Biology: The compound is studied for its antibacterial activity and its potential use in developing new antibiotics.
Medicine: this compound is investigated for its potential therapeutic applications, particularly in treating infections caused by drug-resistant bacteria.
Industry: The compound is used in the development of new antimicrobial agents and as a lead compound for designing new drugs
Mechanism of Action
Pyrrolomycin A exerts its effects by depolarizing the bacterial membrane, leading to the suppression of bacterial bioenergetics through the uncoupling of oxidative phosphorylation. This protonophoric action disrupts the proton gradient across the bacterial membrane, ultimately leading to bacterial cell death. The molecular targets involved in this process include various components of the bacterial membrane and bioenergetic pathways .
Comparison with Similar Compounds
Pyrrolomycin A is unique among the pyrrolomycins due to its specific halogenation pattern and its potent antibacterial activity. Similar compounds in the pyrrolomycin family include:
Pyrrolomycin B: Similar in structure but with different halogenation, showing activity against Gram-positive bacteria.
Pyrrolomycin C: Another halogenated pyrrole with potent antibacterial activity.
Pyrrolomycin D: Known for its strong protonophoric activity and ability to disrupt bacterial membranes
This compound stands out due to its broad-spectrum activity and its potential for further chemical modification to enhance its therapeutic properties.
Biological Activity
Pyrrolomycin A is a member of the pyrrolomycin class of antibiotics, which are known for their potent biological activities, particularly against Gram-positive bacteria. This article delves into the biological activity of this compound, exploring its antimicrobial properties, mechanisms of action, and potential therapeutic applications.
Overview of this compound
This compound is characterized by its polyhalogenated structure, which contributes to its unique biological properties. The compound exhibits significant antibacterial activity, especially against Gram-positive bacteria such as Staphylococcus aureus and Enterococcus faecalis. Its mechanism of action primarily involves disruption of bacterial cell membrane integrity and interference with proton gradients.
Antimicrobial Properties
This compound demonstrates a broad spectrum of antimicrobial activity. Key findings regarding its efficacy include:
- Minimum Inhibitory Concentration (MIC) : this compound shows low MIC values against various bacterial strains, indicating high potency. For example, it has been reported to have an MIC of approximately 1 ng/mL against S. aureus .
- Minimal Bactericidal Concentration (MBC) : The MBC values for this compound are typically in the low microgram range, suggesting effective bactericidal activity when used at appropriate concentrations .
Table 1: Antimicrobial Activity of this compound
Bacterial Strain | MIC (ng/mL) | MBC (µg/mL) |
---|---|---|
Staphylococcus aureus | 1 | 0.5 |
Enterococcus faecalis | 2 | 1 |
Listeria monocytogenes | 3 | 2 |
The mechanism by which this compound exerts its antibacterial effects involves several key processes:
- Membrane Depolarization : Pyrrolomycins act as potent protonophores, leading to membrane depolarization and disruption of the proton motive force essential for ATP synthesis in bacteria . This action is significantly more effective than conventional protonophores like carbonyl cyanide m-chlorophenylhydrazone (CCCP).
- Cell Wall Integrity Disruption : Studies utilizing scanning electron microscopy have shown that treatment with this compound leads to morphological changes in bacterial cells, including blebbing and cell wall rupture, particularly in resistant strains .
- Resistance Mechanisms : Research indicates that resistance to this compound may arise from alterations in drug penetration rather than target modification. Mutants lacking efflux transporters exhibit increased susceptibility to the compound .
Cytotoxicity and Selectivity
Despite its potent antibacterial properties, the cytotoxicity of this compound is a concern for therapeutic applications. However, recent studies have shown that modified analogs exhibit significantly reduced cytotoxicity while maintaining antimicrobial efficacy:
- Selectivity Index : The selectivity index for this compound has been reported to exceed 1000, indicating a favorable safety margin compared to traditional antibiotics like vancomycin .
- Animal Model Studies : While acute toxicity in animal models has been documented (e.g., 20 mg/kg body weight), further research is needed to fully understand the safety profile of this compound and its derivatives .
Case Studies and Research Findings
Several studies have highlighted the potential applications of this compound in treating antibiotic-resistant infections:
- Antibiofilm Activity : Research indicates that Pyrrolomycins possess significant antibiofilm properties against Staphylococcus species, making them promising candidates for treating biofilm-associated infections .
- Combination Therapy : The efficacy of this compound can be enhanced when used in combination with other antibiotics or therapeutic agents, such as mTOR inhibitors, showing potential for synergistic effects in cancer treatment .
Properties
CAS No. |
79763-01-2 |
---|---|
Molecular Formula |
C4H2Cl2N2O2 |
Molecular Weight |
180.97 g/mol |
IUPAC Name |
2,3-dichloro-4-nitro-1H-pyrrole |
InChI |
InChI=1S/C4H2Cl2N2O2/c5-3-2(8(9)10)1-7-4(3)6/h1,7H |
InChI Key |
CDHAYBUDIPNGGJ-UHFFFAOYSA-N |
SMILES |
C1=C(C(=C(N1)Cl)Cl)[N+](=O)[O-] |
Canonical SMILES |
C1=C(C(=C(N1)Cl)Cl)[N+](=O)[O-] |
Key on ui other cas no. |
79763-01-2 |
Synonyms |
pyrrolomycin A SF-2080A |
Origin of Product |
United States |
Retrosynthesis Analysis
AI-Powered Synthesis Planning: Our tool employs the Template_relevance Pistachio, Template_relevance Bkms_metabolic, Template_relevance Pistachio_ringbreaker, Template_relevance Reaxys, Template_relevance Reaxys_biocatalysis model, leveraging a vast database of chemical reactions to predict feasible synthetic routes.
One-Step Synthesis Focus: Specifically designed for one-step synthesis, it provides concise and direct routes for your target compounds, streamlining the synthesis process.
Accurate Predictions: Utilizing the extensive PISTACHIO, BKMS_METABOLIC, PISTACHIO_RINGBREAKER, REAXYS, REAXYS_BIOCATALYSIS database, our tool offers high-accuracy predictions, reflecting the latest in chemical research and data.
Strategy Settings
Precursor scoring | Relevance Heuristic |
---|---|
Min. plausibility | 0.01 |
Model | Template_relevance |
Template Set | Pistachio/Bkms_metabolic/Pistachio_ringbreaker/Reaxys/Reaxys_biocatalysis |
Top-N result to add to graph | 6 |
Feasible Synthetic Routes
Disclaimer and Information on In-Vitro Research Products
Please be aware that all articles and product information presented on BenchChem are intended solely for informational purposes. The products available for purchase on BenchChem are specifically designed for in-vitro studies, which are conducted outside of living organisms. In-vitro studies, derived from the Latin term "in glass," involve experiments performed in controlled laboratory settings using cells or tissues. It is important to note that these products are not categorized as medicines or drugs, and they have not received approval from the FDA for the prevention, treatment, or cure of any medical condition, ailment, or disease. We must emphasize that any form of bodily introduction of these products into humans or animals is strictly prohibited by law. It is essential to adhere to these guidelines to ensure compliance with legal and ethical standards in research and experimentation.