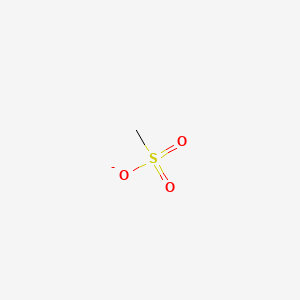
Methanesulfonate
Overview
Description
Methanesulfonate (CH₃SO₃⁻) is the conjugate base of methanesulfonic acid (MSA), a strong organic acid with the formula CH₃SO₃H. This compound salts, also known as mesylates, exhibit unique properties such as high solubility in aqueous solutions, thermal stability, and low corrosivity compared to inorganic acids like sulfuric or hydrochloric acid . These salts are widely used in industrial processes, including hydrometallurgy, catalysis, and pharmaceutical synthesis . This compound is also an environmental marker, serving as a tracer for phytoplankton blooms in atmospheric aerosols .
Scientific Research Applications
Electrochemical Applications
Electrolytes in Energy Storage
Methanesulfonic acid is utilized as an electrolyte in electrochemical systems due to its high solubility, conductivity, and electrochemical stability. MSA-based electrolytes are particularly beneficial in:
- Redox Flow Batteries (RFBs) : MSA offers a safer alternative to traditional electrolytes based on fluoroboric or fluorosilicic acid, enhancing the safety profile of energy storage systems .
- Electrodeposition Processes : MSA is employed in the electrodeposition of tin-lead solder for electronics and high-speed plating of tin on steel plates used in food cans. These processes benefit from MSA's ability to provide a stable and effective plating environment .
Table 1: Comparison of MSA with Traditional Electrolytes
Property | Methanesulfonic Acid | Fluoroboric Acid | Fluorosilicic Acid |
---|---|---|---|
Solubility | High | Moderate | Moderate |
Conductivity | High | High | High |
Environmental Safety | Safer | Less safe | Less safe |
Application | Electroplating, RFBs | Electroplating | Electroplating |
Pharmaceutical Applications
Formation of Sulfonate Esters
Methanesulfonic acid serves as a precursor for the synthesis of methyl methanesulfonate (MMS), which is used in pharmaceutical manufacturing. MMS acts as a reagent in alkylation and esterification reactions, facilitating the modification of active pharmaceutical ingredients (APIs) .
- Regulatory Concerns : The presence of sulfonate esters raises regulatory issues regarding potential genotoxic impurities. Research indicates that conditions such as temperature and the presence of bases can significantly influence the formation of these esters, allowing for better control during API processing .
Table 2: Conditions Affecting MMS Formation
Condition | Effect on MMS Formation |
---|---|
Temperature | Lower temperatures reduce formation |
Water Presence | Increased water content enhances formation |
Base Addition | Excess base prevents formation |
Environmental Remediation
Wastewater Treatment
Recent studies have highlighted the potential of methanesulfonic acid in wastewater treatment processes. Its ability to form complexes with various ions facilitates the extraction and recovery of valuable metals from wastewater streams .
- Reactive Extraction Techniques : The complexation between methanesulfonic acid and other compounds has shown promise in enhancing extraction efficiencies, thereby contributing to more sustainable metal recovery methods .
Toxicological Studies
Carcinogenic Potential
While this compound compounds like methyl this compound are useful in various applications, they also pose health risks. Research has classified methyl this compound as reasonably anticipated to be a human carcinogen based on sufficient evidence from animal studies .
Chemical Reactions Analysis
Nucleophilic Substitution Reactions
Methanesulfonate esters (mesylates) are pivotal in nucleophilic substitution (Sₙ2) reactions due to the excellent leaving-group ability of the mesylate anion (CH₃SO₃⁻).
-
Example Reaction :
Formation of methyl this compound (MMS) via acid-catalyzed esterification:Under anhydrous conditions with 1 M methanesulfonic acid (MSA) in methanol, MMS formation peaks at 0.35% yield at 70°C .
-
Effect of Base :
Adding 2,6-lutidine (a weak base) reduces MMS formation by neutralizing excess acid, suppressing protonation of the sulfonate anion and limiting esterification .
Table 1: Rate Constants for MMS Formation in Methanol
Temperature (°C) | Rate Constant (h⁻¹) |
---|---|
40 | 0.008 |
60 | 0.022 |
70 | 0.035 |
Elimination Reactions
This compound esters undergo base-induced elimination to form alkenes or alkynes.
-
Example Reaction :
But-3-yn-1-yl this compound eliminates in the presence of NaH to yield but-3-yne:
Oxidation Reactions
This compound salts react with hydroxyl radicals (- OH) in atmospheric aerosols, leading to fragmentation:
-
Heterogeneous Oxidation :
Sodium this compound (CH₃SO₃Na) reacts with - OH at 90% relative humidity, producing formaldehyde (HCHO) and sulfite radicals (SO₃- ⁻) :The effective OH uptake coefficient for CH₃SO₃Na is 0.20 ± 0.06, slower than free MSA (0.45 ± 0.14) due to water-layer shielding .
Table 2: OH Oxidation Kinetics
Compound | Effective OH Uptake Coefficient |
---|---|
MSA | 0.45 ± 0.14 |
Sodium this compound | 0.20 ± 0.06 |
Alkylation Reactions
This compound esters act as alkylating agents in biochemical systems:
-
DNA Alkylation :
Ethyl this compound (EMS) transfers ethyl groups to guanine bases in DNA, forming O⁶-ethylguanine adducts, which induce mutations .
Table 3: Mutagenic Activity of EMS
Organism | Mutation Frequency (per 10⁶ cells) |
---|---|
Drosophila | 12.3 |
Human lymphocytes | 8.7 |
Solvolysis Reactions
This compound esters hydrolyze in aqueous or alcoholic media via Sₙ1 or Sₙ2 mechanisms:
-
Kinetic Study :
Solvolysis of methyl this compound in methanol follows first-order kinetics with an activation energy () of 85.6 kJ/mol .
Table 4: Solvolysis Rate Constants
Solvent | Temperature (°C) | Rate Constant (s⁻¹) |
---|---|---|
Methanol | 60 | 1.2 × 10⁻⁴ |
Ethanol | 70 | 3.8 × 10⁻⁴ |
Coordination with Metal Ions
Methanesulfonic acid (MSA) dissolves metal salts efficiently, forming stable complexes:
-
Electroplating Applications :
MSA-based electrolytes enable high-concentration Sn²⁺ and Pb²⁺ solutions for tin-lead alloy electrodeposition, replacing toxic fluoroboric acid .
Radical Chain Reactions
Sulfite radicals (SO₃- ⁻) from this compound oxidation initiate chain reactions in aerosols:
Q & A
Basic Research Questions
Q. What validated analytical methods are recommended for quantifying methanesulfonate derivatives in biological matrices?
Methodological Answer: Gas chromatography-mass spectrometry (GC-MS) with electron capture detection is widely validated for alkyl methanesulfonates (e.g., methyl, ethyl derivatives). Key validation parameters include:
- Linearity : Achieve over 0.4–10 µg/g ranges .
- Precision : System precision should be ≤5% RSD (e.g., 3.82% for methyl this compound) .
- Recovery : Spike-and-recovery experiments should yield 82–97% recovery rates in complex matrices (Table 2 in ).
- Stability : Solutions remain stable for ≤5 hours at 10°C; prolonged storage requires inert atmospheres .
Q. How should researchers design experiments to assess this compound-induced genotoxicity?
Methodological Answer:
- In vitro : Use Ames tests with Salmonella typhimurium strains (TA98, TA100) to detect frameshift/base-pair mutations .
- In vivo : Employ Drosophila melanogaster germline assays (e.g., dominant lethal tests) with dose ranges of 10–50 mM EMS, monitoring embryonic lethality .
- Confounding Control : Include negative controls (solvent-only) and positive controls (e.g., N-ethyl-N-nitrosourea) to validate assay sensitivity .
Q. What are the critical stability considerations for this compound storage in laboratory settings?
Methodological Answer:
- Degradation : this compound esters hydrolyze in aqueous media; store under anhydrous conditions (e.g., desiccated, argon-purged vials) .
- Temperature : Avoid temperatures >25°C; decomposition accelerates at 30°C, releasing mutagenic sulfonic acids .
- Container Material : Use glass or PTFE-lined containers; avoid PVC due to plasticizer leaching .
Advanced Research Questions
Q. How can researchers optimize detection limits for trace this compound contaminants in environmental samples?
Methodological Answer:
- Preconcentration : Solid-phase extraction (SPE) with C18 cartridges improves LOD to 0.3 µg/g .
- Matrix Interference : Use isotope dilution (e.g., -labeled EMS) to correct for ion suppression in GC-MS .
- Ruggedness Testing : Validate across columns (e.g., DB-5 vs. HP-1) to ensure %RSD <5% under varied conditions .
Q. How to reconcile contradictory data on this compound toxicity across in vitro and in vivo models?
Methodological Answer:
- Metabolic Activation : In vitro systems often lack liver S9 fractions, underestimating protoxican activation (e.g., lead this compound’s carcinogenicity is evident only in metabolically competent models) .
- Dose-Response : Apply Hill slope models to non-linear data; low-dose hormesis may obscure linear no-threshold trends .
- Species Sensitivity : Drosophila exhibits 10x higher EMS mutagenicity than rodents due to differential DNA repair efficiency .
Q. What mechanistic insights explain this compound’s alkylation specificity in DNA adduct formation?
Methodological Answer:
- Reactive Sites : EMS preferentially alkylates guanine at N7 positions (70% adducts) versus adenine N3 (20%) due to electron density and steric accessibility (Figure 1 in ).
- pH Dependence : Alkylation peaks at pH 7.2–7.4; acidic conditions protonate sulfonate leaving groups, reducing reactivity .
- Repair Pathways : MGMT (O6-methylguanine methyltransferase) repairs >90% of O6-alkylguanine adducts, but N7 lesions persist, causing replication errors .
Q. How to model this compound ecotoxicology in aquatic systems using ionomics datasets?
Methodological Answer:
- Bioaccumulation : Use ICP-MS to quantify lead this compound in Arabidopsis shoots (PiiMS database: 1M+ elemental concentrations) .
- Trophic Transfer : Apply allometric scaling (log Kow = 1.2) to predict biomagnification in fish; validate via LC50 assays (e.g., 2.1 mg/L for Daphnia magna) .
- Soil Retention : Calculate partition coefficients (Kd) using batch equilibrium tests; methanesulfonates show low adsorption (Kd <10 L/kg) in sandy soils .
Q. Data Contradiction & Synthesis
Q. How should researchers address discrepancies in reported this compound carcinogenicity thresholds?
Methodological Answer:
- Confounder Adjustment : Stratify data by exposure duration (acute vs. chronic) and route (oral vs. dermal). IARC classifies lead this compound as Group 2A (probable human carcinogen), while ethyl derivatives are Group 1 .
- Meta-Analysis : Pool data using random-effects models; heterogeneity indices () >50% indicate need for subgroup analysis (e.g., species, sex) .
Q. Tables for Reference
Parameter | Methyl this compound | Ethyl this compound | Source |
---|---|---|---|
LOD (µg/g) | 0.3 | 0.3 | Table 1 |
LOQ (µg/g) | 0.4 | 0.4 | Table 1 |
Ames Test EC50 (µM) | 120 | 85 | |
Aquatic LC50 (mg/L) | 4.5 | 3.8 |
Comparison with Similar Compounds
Methanesulfonate’s properties and applications are best understood when contrasted with structurally or functionally related compounds. Below is a detailed comparison:
Sulfonic Acids: Methanesulfonic Acid vs. p-Toluenesulfonic Acid (PTSA)
Methanesulfonic acid (MSA) and p-toluenesulfonic acid (PTSA) are both strong sulfonic acids, but their performance varies in industrial applications:
- Leaching Efficiency : MSA outperforms PTSA and HCl in extracting rare earth elements (REEs) from phosphogypsum due to the higher solubility and stability of REE-methanesulfonate complexes. For example, the solubility of REE-methanesulfonates is enhanced by the absence of steric hindrance from aromatic groups (present in PTSA) .
- Environmental Impact : MSA is less toxic and more biodegradable than PTSA, making it preferable for green chemistry applications .
Table 1: Leaching Efficiency of Sulfonic Acids
Leaching Agent | REE Extraction Efficiency | Complex Stability |
---|---|---|
MSA | Highest | Most stable |
PTSA | Moderate | Less stable |
HCl | Lowest | Least stable |
Inorganic Salts: Methanesulfonates vs. Sulfates/Chlorides
Metal this compound salts generally exhibit higher solubility than sulfates but variable solubility compared to chlorides:
Table 2: Solubility of Metal Salts in Water
Metal Ion | This compound (g/L) | Sulfate (g/L) | Chloride (g/L) |
---|---|---|---|
Pb²⁺ | 539 (as metal) | <100 | <100 |
Ag⁺ | >500 | <200 | <300 |
Zn²⁺ | ~300 | <200 | ~450 |
- Exceptions : Zinc chloride (ZnCl₂) is more soluble than zinc this compound, likely due to differences in ionic radius and hydration energy .
Alkylating Agents: this compound Esters
Methyl this compound (CH₃SO₃CH₃, MMS) and ethyl this compound (EMS) are alkylating agents used in mutagenicity studies and drug synthesis:
- Mutagenicity : MMS induces DNA damage by methylating guanine bases, leading to base-pair mismatches. Studies show it increases revertant colonies in Salmonella assays (mutagenicity index: >5) .
- Pharmaceutical Applications: MMS and EMS are monitored as genotoxic impurities in drugs. Analytical methods like HPLC and GC-MS are employed for their detection .
Table 3: Toxicity Profile of Alkylating Agents
Compound | Mutagenicity Index | Primary Use |
---|---|---|
Methyl this compound | >5 | DNA alkylation studies |
Ethyl this compound | >4 | Pharmaceutical impurity testing |
Catalytic Salts: Stannous this compound vs. Other Catalysts
Stannous this compound (Sn(CH₃SO₃)₂) is a highly efficient catalyst in esterification and polymerization reactions:
- Advantages : It offers higher catalytic activity and lower toxicity than traditional catalysts like sulfuric acid or tin chloride .
- Market Growth: The global stannous this compound market is projected to expand due to demand in fragrances, resins, and fine chemical synthesis .
Rare Earth Methanesulfonates
Gadolinium and lutetium methanesulfonates are studied for their thermal stability and coordination chemistry. Thermogravimetric analysis (TGA) reveals decomposition temperatures exceeding 300°C, making them suitable for high-temperature applications .
Key Research Findings
Hydrometallurgy : MSA’s high leaching efficiency for REEs is attributed to the formation of stable, water-soluble complexes .
Environmental Chemistry : this compound’s role as a phytoplankton bloom marker underscores its ecological significance .
Limitations : Solubility data for methanesulfonates are incomplete, particularly for temperature-dependent behavior .
Preparation Methods
Industrial Preparation via Metal Catalysts and Replacement Reactions
Industrial-scale methanesulfonate production often leverages metal catalysts, particularly for generating tin this compound, a valuable electroplating agent. A patented method (CN105061269A) demonstrates the recycling of spent methylsulfonate catalysts into high-purity tin this compound through a four-step replacement reaction .
Catalyst Purification and Organic Matter Removal
The process begins with purifying discarded methylsulfonate catalysts (e.g., copper this compound or silver this compound) using a two-phase separation method. Ethanol washing and evaporation remove residual organic contaminants, yielding anhydrous metal this compound solids. For example, 200 g of spent copper this compound catalyst was purified to 180 g of organic-free solid .
Solution Preparation and Stabilization
The purified metal this compound is dissolved in deionized water to form a 20–45 wt% solution. To prevent tin oxidation and solution discoloration, 5–10 wt% MSA and 0.25–1 wt% resorcinol (as an anti-oxidant) are added. This stabilizes the solution for subsequent metallic tin substitution .
Replacement Reaction and Product Isolation
Metallic tin granules react with the metal this compound solution under vacuum agitation. The replacement reaction displaces less active metals (e.g., copper or silver) from the this compound complex, forming tin this compound and precipitating noble metal powders. In a representative trial, 200 g of tin reacted with copper this compound solution for 24 hours, yielding 408 g of 50.2 wt% tin this compound solution and 43.6 g of copper powder .
Table 1: Industrial Tin this compound Synthesis Parameters
Parameter | Example 1 | Example 2 | Example 3 |
---|---|---|---|
Catalyst Input (g) | 200 Cu | 300 Cu | 220 Ag |
Tin Input (g) | 200 | 300 | — |
Reaction Time (hr) | 24 | 20 | 18 |
Product Yield (g) | 408 | 571 | 306 |
Purity (wt%) | 50.2 | 50.1 | 50.2 |
Hydrothermal Synthesis of Mixed-Anionic Methanesulfonates
Recent advances in materials science have enabled the synthesis of mixed-anionic sodium methanesulfonates with tunable properties. A 2025 study demonstrated the preparation of Na₅(SO₃CH₃)₄(X) (X = BF₄⁻, ClO₄⁻, PF₆⁻, I⁻) through hydrothermal methods .
Structural Engineering via Anion Substitution
The parent compound, NaSO₃CH₃, features [Na₅(SO₃CH₃)₄]ⁿ⁺ layers intercalated with this compound anions. By substituting the interlayer anion with BF₄⁻, ClO₄⁻, PF₆⁻, or I⁻ during crystallization, researchers achieved isostructural derivatives with distinct thermal and optical properties .
Crystal Growth and Stability
Compounds with BF₄⁻, ClO₄⁻, and PF₆⁻ anions formed centimeter-sized crystals under ambient conditions, while the iodide variant required hydrothermal treatment at 120°C. Thermogravimetric analysis revealed decomposition temperatures ranging from 420°C (ClO₄⁻) to 490°C (BF₄⁻), with residual products including NaF, NaCl, and Na₂CO₃ depending on the anion .
Pharmaceutical Context: Controlling Genotoxic this compound Esters
This compound esters like methyl this compound (MMS) and ethyl this compound (EMS) are potent genotoxins that can form during drug synthesis. A 2022 study established a derivatization HPLC method to quantify these impurities at ppm levels .
Ester Formation Mechanisms
MSA reacts spontaneously with short-chain alcohols (e.g., methanol, ethanol) in API synthesis. For example, the drug IMH (a morpholine methylbiphenyl this compound) risks forming MMS if residual methanol remains during salt formation .
Analytical Control Strategies
Key parameters for impurity suppression include:
-
pH Control : NaOH (40 mg/mL) optimizes derivatization efficiency while avoiding matrix interference .
-
Temperature : Heating at 80°C for 60 minutes maximizes reaction yields without degrading APIs .
-
Chromatography : ACN/5 mM ammonium acetate mobile phase on a C18 column resolves MMS/EMS derivatives from drug components .
Acid-Catalyzed Esterification: Mechanistic Insights
The formation of methyl this compound (MMS) from MSA and methanol follows an acid-catalyzed bimolecular nucleophilic substitution (SN2) mechanism. Isotopic labeling studies using ¹⁸O-methanol confirmed that the sulfonate oxygen originates from methanol rather than MSA .
Reaction Kinetics and Optimization
-
Catalyst : Lutidine this compound (1 M in methanol) accelerates esterification at 40–70°C .
-
Equilibrium : High alcohol concentrations favor ester formation, while water drives hydrolysis.
-
Side Reactions : Prolonged heating above 80°C promotes sulfonic anhydride formation, necessitating precise temperature control .
Table 2: MMS Synthesis Conditions and Yields
Condition | Optimal Range | Effect on Yield |
---|---|---|
Temperature | 60–70°C | Maximizes rate |
MSA:Methanol Ratio | 1:3 molar | Shifts equilibrium |
Reaction Time | 2–4 hours | Prevents degradation |
Comparative Analysis of Synthesis Methods
Scalability and Cost
-
Industrial Catalytic Method : High throughput (kg-scale) but requires noble metal recovery .
-
Hydrothermal Synthesis : Suitable for specialty materials but limited to gram-scale .
-
Pharmaceutical-Grade : Prioritizes purity over yield, with stringent impurity controls .
Environmental Impact
The catalytic replacement method reduces waste by converting spent catalysts into usable products (87% copper recovery) . In contrast, traditional esterification generates stoichiometric acidic byproducts requiring neutralization .
Properties
CAS No. |
16053-58-0 |
---|---|
Molecular Formula |
CH3O3S- |
Molecular Weight |
95.1 g/mol |
IUPAC Name |
methanesulfonate |
InChI |
InChI=1S/CH4O3S/c1-5(2,3)4/h1H3,(H,2,3,4)/p-1 |
InChI Key |
AFVFQIVMOAPDHO-UHFFFAOYSA-M |
SMILES |
CS(=O)(=O)[O-] |
Canonical SMILES |
CS(=O)(=O)[O-] |
Synonyms |
arium methanesulfonate BMS-480188 methanesulfonate methanesulfonic acid methanesulfonic acid, ammonia salt methanesulfonic acid, chromium (2+) salt methanesulfonic acid, chromium (3+) salt methanesulfonic acid, cobalt (2+) salt methanesulfonic acid, copper (2+) salt methanesulfonic acid, iron (2+) salt methanesulfonic acid, iron (3+)salt methanesulfonic acid, nickel (2+) salt methanesulfonic acid, potassium salt methanesulfonic acid, silver (1+) salt methanesulfonic acid, sodium salt methylsulfonate potassium mesylate potassium methanesulfonate |
Origin of Product |
United States |
Synthesis routes and methods I
Procedure details
Synthesis routes and methods II
Procedure details
Synthesis routes and methods III
Procedure details
Synthesis routes and methods IV
Procedure details
Synthesis routes and methods V
Procedure details
Retrosynthesis Analysis
AI-Powered Synthesis Planning: Our tool employs the Template_relevance Pistachio, Template_relevance Bkms_metabolic, Template_relevance Pistachio_ringbreaker, Template_relevance Reaxys, Template_relevance Reaxys_biocatalysis model, leveraging a vast database of chemical reactions to predict feasible synthetic routes.
One-Step Synthesis Focus: Specifically designed for one-step synthesis, it provides concise and direct routes for your target compounds, streamlining the synthesis process.
Accurate Predictions: Utilizing the extensive PISTACHIO, BKMS_METABOLIC, PISTACHIO_RINGBREAKER, REAXYS, REAXYS_BIOCATALYSIS database, our tool offers high-accuracy predictions, reflecting the latest in chemical research and data.
Strategy Settings
Precursor scoring | Relevance Heuristic |
---|---|
Min. plausibility | 0.01 |
Model | Template_relevance |
Template Set | Pistachio/Bkms_metabolic/Pistachio_ringbreaker/Reaxys/Reaxys_biocatalysis |
Top-N result to add to graph | 6 |
Feasible Synthetic Routes
Disclaimer and Information on In-Vitro Research Products
Please be aware that all articles and product information presented on BenchChem are intended solely for informational purposes. The products available for purchase on BenchChem are specifically designed for in-vitro studies, which are conducted outside of living organisms. In-vitro studies, derived from the Latin term "in glass," involve experiments performed in controlled laboratory settings using cells or tissues. It is important to note that these products are not categorized as medicines or drugs, and they have not received approval from the FDA for the prevention, treatment, or cure of any medical condition, ailment, or disease. We must emphasize that any form of bodily introduction of these products into humans or animals is strictly prohibited by law. It is essential to adhere to these guidelines to ensure compliance with legal and ethical standards in research and experimentation.