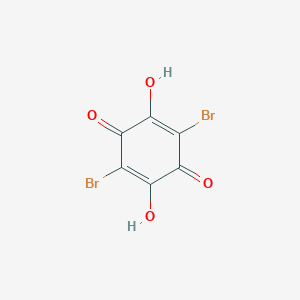
Bromanilic acid
Overview
Description
Bromanilic acid (BRA), chemically designated as 2,5-dibromo-3,6-dihydroxy-p-benzoquinone (C₆H₂Br₂O₄, CAS 4370-59-6), is a halogenated derivative of 3,6-dihydroxy-p-benzoquinone. Its structure features two bromine atoms at the 2- and 5-positions and hydroxyl groups at the 3- and 6-positions, forming a planar aromatic system . This compound is widely used in coordination chemistry and crystal engineering due to its ability to act as a hydrogen bond donor/acceptor and participate in charge-transfer interactions .
Preparation Methods
Crystallographic Characterization of Bromanilic Acid Derivatives
Morpholinium Hydrogen Bromanilate: A Case Study
Single crystals of morpholinium hydrogen bromanilate (C₄H₁₀NO⁺·C₆HBr₂O₄⁻) were obtained by slow evaporation of an acetonitrile solution containing this compound and morpholine . The crystal structure, resolved at 130 K, reveals a monoclinic lattice (space group P2₁/c) with unit cell parameters a = 12.907 Å, b = 7.274 Å, c = 13.125 Å, and β = 91.95° . Key bond lengths and angles are summarized below:
Parameter | Value |
---|---|
Br1–C2 bond length | 1.897 Å |
O2–H2···O4 hydrogen bond | 1.79 Å (H···O) |
O4–H4···O2 hydrogen bond | 1.80 Å (H···O) |
C6–O4–C4 dihedral angle | 123.69° |
The hydrogen-bonding network features disordered protons in short O–H···O interactions (2.52 Å), with occupancy factors of 0.50 . This disorder, attributed to proton tautomerism, aligns with observations in analogous chloranilate systems .
Hydrogen-Bonding and Thermal Stability
Temperature-dependent studies of morpholinium hydrogen bromanilate reveal anomalous behavior in hydrogen-bond dynamics. Nuclear quadrupole resonance (NQR) data indicate that one chlorine site exhibits frequency increases with temperature, contrasting conventional lattice thermal expansion models . This anomaly correlates with structural flexibility in the O–H···O framework, as confirmed by multi-temperature X-ray diffraction .
Purification and Crystallization Techniques
Solvent Selection and Evaporation
The crystallization of this compound derivatives requires solvents capable of stabilizing ionic hydrogen bonds. Acetonitrile, used in the preparation of morpholinium hydrogen bromanilate, facilitates slow evaporation and high-quality crystal growth . Polar aprotic solvents (e.g., DMF, DMSO) are avoided due to competitive coordination with bromanilate anions.
Mechanistic Insights into Bromination and Esterification
Bromination Kinetics
In the synthesis of bromoacetic acid, the addition of concentrated sulfuric acid to chloroacetic acid and KBr proceeds exothermically, requiring temperature control (40–70°C) to prevent side reactions . A molar ratio of 1:1.3:1.1 (chloroacetic acid:KBr:H₂SO₄) optimizes bromide ion activity while minimizing sulfonation . Similar principles likely govern the bromination of quinone substrates.
Esterification Pathways
Esterification of this compound with alcohols (e.g., methanol, ethanol) proceeds via acid-catalyzed nucleophilic acyl substitution. The patent-described two-step process—bromination followed by esterification—could be adapted for this compound esters by substituting chloroacetic acid with chloranilic acid .
Applications and Functional Derivatives
Coordination Polymers
This compound’s ability to act as a ditopic ligand enables the construction of metal-organic frameworks (MOFs). The morpholinium salt’s disordered hydrogen bonds suggest potential for proton-conductive materials .
Redox-Active Materials
The quinoid core of this compound supports reversible electron transfer, making it suitable for organic battery electrolytes or electrochromic devices. Functionalization with electron-donating groups (e.g., –NH₂, –OCH₃) could modulate its redox potential.
Chemical Reactions Analysis
Types of Reactions: Bromanilic acid undergoes various chemical reactions, including:
Oxidation: this compound can be oxidized to form brominated quinones.
Reduction: It can be reduced to form hydroquinone derivatives.
Substitution: The bromine atoms in this compound can be substituted with other functional groups under appropriate conditions.
Common Reagents and Conditions:
Oxidation: Common oxidizing agents include potassium permanganate and hydrogen peroxide.
Reduction: Reducing agents such as sodium borohydride and lithium aluminum hydride are used.
Substitution: Nucleophiles like amines and thiols can replace bromine atoms in the presence of catalysts.
Major Products:
Oxidation: Brominated quinones.
Reduction: Hydroquinone derivatives.
Substitution: Various substituted quinones depending on the nucleophile used.
Scientific Research Applications
Chemical Applications
Synthesis of Brominated Compounds
Bromanilic acid serves as a precursor in the synthesis of other brominated compounds. Its unique structure allows it to participate in various chemical reactions, facilitating the production of more complex molecules.
Charge-Assisted Hydrogen Bonding Studies
Research has utilized this compound to investigate charge-assisted hydrogen bonding interactions. These studies are crucial for understanding molecular interactions in various chemical systems, particularly in crystal engineering and material sciences .
Biological Applications
Antimicrobial and Anticancer Properties
Derivatives of this compound are being explored for their potential biological activities. Preliminary studies suggest that these derivatives may exhibit antimicrobial and anticancer properties, making them candidates for further pharmacological research .
Drug Design and Development
Ongoing research is focused on the use of this compound in drug design. Its structural characteristics may contribute to the development of new compounds with improved pharmacological profiles, particularly in targeting specific biological pathways .
Industrial Applications
Production of Dyes and Pigments
In industrial settings, this compound is utilized in the manufacture of dyes and pigments. Its brominated structure imparts unique color properties that are valuable in various applications, including textiles and coatings .
Case Study 1: Crystal Engineering
A study investigated the co-crystallization of this compound with different picolines to examine halogen bonding capabilities. The resulting complexes demonstrated significant hydrogen-bonding interactions, which were analyzed using single-crystal X-ray diffraction techniques. This research provides insights into the structural patterns that emerge from halogen bonding interactions .
Complex | Ratio | Key Findings |
---|---|---|
This compound + 3-Picoline | 1:1 | Observed bifurcated hydrogen bonds |
This compound + 4-Picoline | 1:2 | Enhanced stability due to multiple hydrogen bonding |
Case Study 2: Ferroelectric Properties
Research on supramolecular ferroelectric cocrystals involving this compound has highlighted its potential in electronic applications. The study revealed that these cocrystals exhibit unique ferroelectric properties under varying pressure conditions, indicating their applicability in advanced material science .
Mechanism of Action
The mechanism of action of bromanilic acid involves its ability to form charge-assisted hydrogen bonds. This property is crucial in its interactions with other molecules, influencing their electronic and structural properties . In biological systems, this compound and its derivatives can interact with various molecular targets, potentially affecting cellular processes and pathways.
Comparison with Similar Compounds
Bromanilic acid belongs to the halo-anilic acid family, which includes chloranilic (CLA, Cl-substituted) and iodanilic (IA, I-substituted) acids. These compounds share a common quinoid backbone but differ in halogen substituents, leading to distinct physicochemical and structural behaviors.
Structural Properties and Hydrogen Bonding
- Crystal Packing :
this compound forms 1:1 stoichiometric complexes with bases like 4,4′-di-tert-butyl-2,2′-bipyridyl (dtBBP), analogous to CLA and IA. However, the larger atomic radius of Br (vs. Cl or I) introduces steric and electronic differences. For example, in dtBBP·BRA, the hydrogen bond between N⁺(1)–H(1) and O(5) is weaker than in dtBBP·CLA, evidenced by longer N⁺–H distances (1.30(7) Å vs. 1.05(2) Å) and shorter H···O distances (1.39(7) Å vs. 1.60(2) Å) .- Key Structural Metrics :
Parameter | dtBBP·BRA | dtBBP·CLA |
---|---|---|
N⁺–H distance (Å) | 1.30(7) | 1.05(2) |
H···O distance (Å) | 1.39(7) | 1.60(2) |
∠(N⁺–H···O) | 155° | 160° |
Halogen atomic radius | 1.85 Å (Br) | 0.99 Å (Cl) |
- Halogen Effects: this compound’s larger Br atoms reduce hydrogen bond strength compared to CLA, influencing thermal stability and phase transitions. In contrast, iodanilic acid (IA) exhibits stronger hydrogen bonds due to iodine’s polarizability, leading to higher phase transition temperatures (e.g., 331 K in dtBBP·IA vs. none observed in dtBBP·BRA) .
Thermal and Phase Behavior
- Neat dtBBP exhibits transitions at 165 K and 219 K, attributed to tert-butyl group dynamics, which are less pronounced in BRA complexes due to weaker intermolecular interactions .
Coordination Chemistry and Metal Complexes
- Lanthanoid Coordination: this compound forms isostructural complexes with lanthanoids (e.g., [Ln(BRA)(fma)]), similar to CLA. However, IR spectra reveal distinct C–Br stretching vibrations (~500–600 cm⁻¹) compared to C–Cl (~600–700 cm⁻¹), affecting ligand-field splitting and coordination geometry .
- Mo(VI) Complexes :
In molybdenum hydrazone complexes, this compound (H₂BrA) competes with chloranilic acid (H₂ClA) as counteranions. The larger Br atoms in 1H0.5·2MeOH create different packing arrangements and stabilize distinct supramolecular architectures compared to CLA analogues .
Spectroscopic and Electronic Properties
- Electrostatic Potential: Charge density studies of 2,5-lutidinium bromanilate reveal bifurcated N⁺–H···O hydrogen bonds, with electron density redistributed toward Br atoms. This contrasts with CLA complexes, where electron withdrawal by Cl enhances hydrogen bond acidity .
- Solid-State NMR : Co-crystals of BRA with 2,6-dimethylpyrazine show distinct ¹³C chemical shifts due to Br’s electron-withdrawing effects, altering π-stacking interactions compared to CLA analogues .
Biological Activity
Bromanilic acid, a brominated derivative of vanillic acid, has garnered attention for its diverse biological activities. This article delves into the compound's pharmacological properties, mechanisms of action, and potential therapeutic applications based on recent research findings.
This compound (CHBrO) is characterized by its unique structure, which includes two bromine atoms attached to the benzene ring. This structure contributes to its biological activity and interaction with various biological targets.
Biological Activities
1. Antitumor Activity
Research has indicated that this compound exhibits significant antitumor properties. A study highlighted its ability to induce apoptosis in cancer cell lines, particularly in human epidermoid carcinoma and melanoma cells. The mechanism involves the modulation of cell adhesion molecules and the activation of apoptotic pathways .
2. Antimicrobial Properties
This compound has shown promising antimicrobial activity against several bacterial strains, including Staphylococcus aureus and Candida albicans. Its efficacy as an antimicrobial agent stems from its ability to disrupt bacterial cell membranes and inhibit protein synthesis .
3. Antioxidant Activity
The compound also demonstrates antioxidant properties, which are crucial for mitigating oxidative stress in cells. This compound scavenges free radicals, thereby protecting cells from oxidative damage and potentially reducing the risk of chronic diseases .
The biological effects of this compound can be attributed to several mechanisms:
- Apoptosis Induction : this compound activates caspases, leading to programmed cell death in tumor cells.
- Cell Adhesion Modulation : It affects the expression of adhesion molecules, influencing cancer cell migration and metastasis.
- Antioxidant Activity : By neutralizing free radicals, it helps maintain cellular integrity and function.
Case Study 1: Antitumor Efficacy
A recent study assessed the effects of this compound on H295R adrenocortical carcinoma cells. The compound was found to significantly reduce cell viability in a dose-dependent manner, with IC50 values indicating potent antitumor activity. The study utilized the MTT assay to evaluate cytotoxicity and confirmed apoptosis through flow cytometry analysis.
Concentration (µM) | Cell Viability (%) |
---|---|
0 | 100 |
10 | 85 |
25 | 65 |
50 | 40 |
100 | 15 |
Case Study 2: Antimicrobial Activity
In another investigation, this compound was tested against various pathogens using agar diffusion methods. The results demonstrated a clear zone of inhibition against E. coli and S. aureus, indicating its potential as a therapeutic agent in treating bacterial infections.
Pathogen | Zone of Inhibition (mm) |
---|---|
Staphylococcus aureus | 15 |
Escherichia coli | 12 |
Candida albicans | 10 |
Q & A
Basic Research Questions
Q. What experimental methods are recommended for synthesizing and characterizing bromanilic acid co-crystals?
this compound co-crystals are typically synthesized via solvent evaporation or slow diffusion methods. Characterization involves low-temperature single-crystal X-ray diffraction to resolve protonation states and hydrogen-bonding networks . For vibrational dynamics, combine infrared (IR), Raman spectroscopy, and inelastic neutron scattering (INS) with solid-state DFT calculations to validate harmonic approximations . Thermal stability is assessed using differential scanning calorimetry (DSC) and thermogravimetric analysis (TGA) .
Q. How can hydrogen-bonding interactions in this compound complexes be validated experimentally?
Use high-resolution X-ray diffraction (up to sinθ/λ = 0.94 Å⁻¹) at low temperatures (e.g., 100 K) to capture electron density distribution. Pair this with Hirshfeld surface analysis to quantify intermolecular interactions (e.g., O···H–N bifurcated hydrogen bonds) and electrostatic potential mapping . Complement with solid-state NMR (e.g., ¹³C CP/MAS) to assign crystallographic resonances and confirm hydrogen-bond strength .
Q. What are the primary challenges in crystallizing this compound derivatives, and how can they be addressed?
Key challenges include polymorphism, solvent selection, and controlling proton transfer during co-crystal formation. Design experiments with systematic solvent screening (polar vs. non-polar) and pH adjustments to stabilize charge-assisted hydrogen bonds. Use SHELXL for refinement to resolve disorder or twinning in diffraction data .
Advanced Research Questions
Q. How do bifurcated hydrogen bonds in this compound complexes influence charge transfer and supramolecular assembly?
Bifurcated hydrogen bonds (e.g., N–H···O) facilitate charge transfer between this compound (Lewis acid) and heterocyclic bases (Lewis base), forming ionic molecular complexes. Analyze topology via Quantum Theory of Atoms in Molecules (QTAIM) to identify bond critical points (BCPs) and Laplacian values. Compare electrostatic potential surfaces (EPS) derived from X-ray charge density data to DFT-computed models .
Q. What computational strategies reconcile discrepancies between experimental and theoretical vibrational spectra of this compound co-crystals?
Employ dispersion-corrected solid-state DFT (e.g., PBE-D3) to account for long-range dipole coupling and anharmonic effects. Validate against experimental IR/THz spectra by refining force constants and scaling factors. For unresolved modes, use inelastic neutron scattering (INS) to detect low-frequency vibrations obscured in optical spectra .
Q. How can researchers resolve contradictions in crystallographic data interpretation for this compound systems?
Contradictions often arise from disordered solvent molecules or dynamic proton motion. Apply noncovalent interaction (NCI) analysis to distinguish hydrogen bonds from van der Waals contacts. Cross-validate with ¹³C CP/MAS NMR to confirm protonation states and SHELXD/SHELXE pipelines for high-throughput phasing of ambiguous regions .
Q. What methodologies optimize the extraction of electrostatic parameters for drug design applications involving this compound derivatives?
Use ultra-high-resolution X-ray data (≤ 0.8 Å) to derive one-electron properties (e.g., electrostatic potential, atomic moments). Integrate with docking studies by exporting ESP maps to molecular dynamics (MD) software. Validate against Hirshfeld surface-derived electrostatic contributions to binding energy .
Q. Methodological Guidance for Data Analysis
Q. How should researchers handle incomplete or conflicting datasets in charge density studies?
- Step 1: Apply multipole refinement in SHELXL to model residual density peaks .
- Step 2: Use CrystalExplorer for Hirshfeld surface analysis to identify unaccounted intermolecular interactions .
- Step 3: Cross-reference with solid-state NMR to resolve ambiguities in proton positions .
Q. What criteria distinguish robust vs. unreliable computational models for this compound systems?
Properties
IUPAC Name |
2,5-dibromo-3,6-dihydroxycyclohexa-2,5-diene-1,4-dione | |
---|---|---|
Source | PubChem | |
URL | https://pubchem.ncbi.nlm.nih.gov | |
Description | Data deposited in or computed by PubChem | |
InChI |
InChI=1S/C6H2Br2O4/c7-1-3(9)5(11)2(8)6(12)4(1)10/h9,12H | |
Source | PubChem | |
URL | https://pubchem.ncbi.nlm.nih.gov | |
Description | Data deposited in or computed by PubChem | |
InChI Key |
GMZWPTALVQRAFV-UHFFFAOYSA-N | |
Source | PubChem | |
URL | https://pubchem.ncbi.nlm.nih.gov | |
Description | Data deposited in or computed by PubChem | |
Canonical SMILES |
C1(=C(C(=O)C(=C(C1=O)Br)O)Br)O | |
Source | PubChem | |
URL | https://pubchem.ncbi.nlm.nih.gov | |
Description | Data deposited in or computed by PubChem | |
Molecular Formula |
C6H2Br2O4 | |
Source | PubChem | |
URL | https://pubchem.ncbi.nlm.nih.gov | |
Description | Data deposited in or computed by PubChem | |
DSSTOX Substance ID |
DTXSID1063437 | |
Record name | 2,5-Cyclohexadiene-1,4-dione, 2,5-dibromo-3,6-dihydroxy- | |
Source | EPA DSSTox | |
URL | https://comptox.epa.gov/dashboard/DTXSID1063437 | |
Description | DSSTox provides a high quality public chemistry resource for supporting improved predictive toxicology. | |
Molecular Weight |
297.89 g/mol | |
Source | PubChem | |
URL | https://pubchem.ncbi.nlm.nih.gov | |
Description | Data deposited in or computed by PubChem | |
CAS No. |
4370-59-6 | |
Record name | 2,5-Dibromo-3,6-dihydroxy-2,5-cyclohexadiene-1,4-dione | |
Source | CAS Common Chemistry | |
URL | https://commonchemistry.cas.org/detail?cas_rn=4370-59-6 | |
Description | CAS Common Chemistry is an open community resource for accessing chemical information. Nearly 500,000 chemical substances from CAS REGISTRY cover areas of community interest, including common and frequently regulated chemicals, and those relevant to high school and undergraduate chemistry classes. This chemical information, curated by our expert scientists, is provided in alignment with our mission as a division of the American Chemical Society. | |
Explanation | The data from CAS Common Chemistry is provided under a CC-BY-NC 4.0 license, unless otherwise stated. | |
Record name | 2,5-Dibromo-3,6-dihydroxyquinone | |
Source | ChemIDplus | |
URL | https://pubchem.ncbi.nlm.nih.gov/substance/?source=chemidplus&sourceid=0004370596 | |
Description | ChemIDplus is a free, web search system that provides access to the structure and nomenclature authority files used for the identification of chemical substances cited in National Library of Medicine (NLM) databases, including the TOXNET system. | |
Record name | Bromanilic acid | |
Source | DTP/NCI | |
URL | https://dtp.cancer.gov/dtpstandard/servlet/dwindex?searchtype=NSC&outputformat=html&searchlist=36928 | |
Description | The NCI Development Therapeutics Program (DTP) provides services and resources to the academic and private-sector research communities worldwide to facilitate the discovery and development of new cancer therapeutic agents. | |
Explanation | Unless otherwise indicated, all text within NCI products is free of copyright and may be reused without our permission. Credit the National Cancer Institute as the source. | |
Record name | 2,5-Cyclohexadiene-1,4-dione, 2,5-dibromo-3,6-dihydroxy- | |
Source | EPA Chemicals under the TSCA | |
URL | https://www.epa.gov/chemicals-under-tsca | |
Description | EPA Chemicals under the Toxic Substances Control Act (TSCA) collection contains information on chemicals and their regulations under TSCA, including non-confidential content from the TSCA Chemical Substance Inventory and Chemical Data Reporting. | |
Record name | 2,5-Cyclohexadiene-1,4-dione, 2,5-dibromo-3,6-dihydroxy- | |
Source | EPA DSSTox | |
URL | https://comptox.epa.gov/dashboard/DTXSID1063437 | |
Description | DSSTox provides a high quality public chemistry resource for supporting improved predictive toxicology. | |
Record name | Bromanilic Acid | |
Source | European Chemicals Agency (ECHA) | |
URL | https://echa.europa.eu/information-on-chemicals | |
Description | The European Chemicals Agency (ECHA) is an agency of the European Union which is the driving force among regulatory authorities in implementing the EU's groundbreaking chemicals legislation for the benefit of human health and the environment as well as for innovation and competitiveness. | |
Explanation | Use of the information, documents and data from the ECHA website is subject to the terms and conditions of this Legal Notice, and subject to other binding limitations provided for under applicable law, the information, documents and data made available on the ECHA website may be reproduced, distributed and/or used, totally or in part, for non-commercial purposes provided that ECHA is acknowledged as the source: "Source: European Chemicals Agency, http://echa.europa.eu/". Such acknowledgement must be included in each copy of the material. ECHA permits and encourages organisations and individuals to create links to the ECHA website under the following cumulative conditions: Links can only be made to webpages that provide a link to the Legal Notice page. | |
Retrosynthesis Analysis
AI-Powered Synthesis Planning: Our tool employs the Template_relevance Pistachio, Template_relevance Bkms_metabolic, Template_relevance Pistachio_ringbreaker, Template_relevance Reaxys, Template_relevance Reaxys_biocatalysis model, leveraging a vast database of chemical reactions to predict feasible synthetic routes.
One-Step Synthesis Focus: Specifically designed for one-step synthesis, it provides concise and direct routes for your target compounds, streamlining the synthesis process.
Accurate Predictions: Utilizing the extensive PISTACHIO, BKMS_METABOLIC, PISTACHIO_RINGBREAKER, REAXYS, REAXYS_BIOCATALYSIS database, our tool offers high-accuracy predictions, reflecting the latest in chemical research and data.
Strategy Settings
Precursor scoring | Relevance Heuristic |
---|---|
Min. plausibility | 0.01 |
Model | Template_relevance |
Template Set | Pistachio/Bkms_metabolic/Pistachio_ringbreaker/Reaxys/Reaxys_biocatalysis |
Top-N result to add to graph | 6 |
Feasible Synthetic Routes
Disclaimer and Information on In-Vitro Research Products
Please be aware that all articles and product information presented on BenchChem are intended solely for informational purposes. The products available for purchase on BenchChem are specifically designed for in-vitro studies, which are conducted outside of living organisms. In-vitro studies, derived from the Latin term "in glass," involve experiments performed in controlled laboratory settings using cells or tissues. It is important to note that these products are not categorized as medicines or drugs, and they have not received approval from the FDA for the prevention, treatment, or cure of any medical condition, ailment, or disease. We must emphasize that any form of bodily introduction of these products into humans or animals is strictly prohibited by law. It is essential to adhere to these guidelines to ensure compliance with legal and ethical standards in research and experimentation.