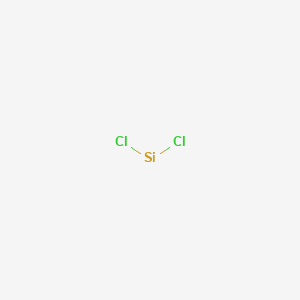
Dichlorosilylene
Overview
Description
Dichlorosilylene, also known as trichlorosilane, is a chemical compound with the formula HSiCl₃. It is a colorless, volatile liquid that is highly reactive and used extensively in the production of high-purity silicon and various silicon-based compounds. Trichlorosilane is a key intermediate in the semiconductor industry and plays a crucial role in the manufacturing of silicon wafers and photovoltaic cells.
Mechanism of Action
Target of Action
Dichlorosilylene, also known as silicon dichloride or silicon chloride hydride, primarily targets the divalent silicon center . The reactivity of this compound is governed by the substituents at this center .
Mode of Action
This compound exhibits ambiphilic behavior, similar to carbenes . It manifests dual-type reactivity:
- Electrophilic : As electron pair acceptors, the acceptor orbital is a silicon 3p-orbital (Lewis acid reactivity) .
- Nucleophilic : As electron pair donors, the donor orbital is a silicon lone pair n-orbital (Lewis base reactivity) .
Biochemical Pathways
The biochemical pathways of this compound involve the interaction of the compound with its targets, leading to various reactions. For instance, this compound can undergo reactions involving the attack of the vacant p-orbital of the silicon center by a Lewis basic reagent .
Pharmacokinetics
It’s important to note that the compound’s reactivity is influenced by the substituents at the divalent silicon center .
Result of Action
The result of this compound’s action depends on its interaction with its targets. For example, it can lead to the formation of novel unsaturated four-membered ring disilene .
Action Environment
The action of this compound is influenced by environmental factors. For instance, σ-electron-withdrawing substituents (such as halogens) promote preferentially electrophilic behavior of silylenes, whereas π-electron-donating groups (such as amino-substituents) favor nucleophilic reactivity of silylenes .
Preparation Methods
Synthetic Routes and Reaction Conditions: Trichlorosilane is typically synthesized through the reaction of silicon with hydrogen chloride gas. The reaction is carried out at elevated temperatures, usually around 300°C, in the presence of a catalyst such as copper: [ \text{Si} + 3 \text{HCl} \rightarrow \text{HSiCl}_3 + \text{H}_2 ]
Industrial Production Methods: In industrial settings, trichlorosilane is produced in large quantities using fluidized bed reactors. Silicon is reacted with hydrogen chloride gas in the presence of a catalyst, and the resulting trichlorosilane is purified through distillation to remove impurities. This high-purity trichlorosilane is then used in the production of polycrystalline silicon for the semiconductor and solar industries .
Chemical Reactions Analysis
Types of Reactions: Trichlorosilane undergoes various chemical reactions, including:
Reduction: Trichlorosilane can be reduced to silane (SiH₄) using lithium aluminium hydride: [ \text{HSiCl}_3 + \text{LiAlH}_4 \rightarrow \text{SiH}_4 + \text{LiCl} + \text{AlCl}_3 ]
Hydrolysis: Trichlorosilane reacts with water to form silicon dioxide and hydrochloric acid: [ \text{HSiCl}_3 + 2 \text{H}_2\text{O} \rightarrow \text{SiO}_2 + 3 \text{HCl} ]
Alcoholysis: Trichlorosilane reacts with alcohols to form alkoxysilanes and hydrochloric acid: [ \text{HSiCl}_3 + 3 \text{ROH} \rightarrow \text{HSi(OR)}_3 + 3 \text{HCl} ]
Common Reagents and Conditions: Common reagents used in reactions with trichlorosilane include lithium aluminium hydride for reduction, water for hydrolysis, and alcohols for alcoholysis. These reactions are typically carried out under controlled conditions to ensure safety and efficiency.
Major Products: The major products formed from these reactions include silane, silicon dioxide, alkoxysilanes, and hydrochloric acid .
Scientific Research Applications
Trichlorosilane has a wide range of applications in scientific research and industry:
Chemistry: It is used as a precursor for the synthesis of various organosilicon compounds and as a reducing agent in organic synthesis.
Biology: Trichlorosilane-modified surfaces are used in biochip technology and biosensors.
Medicine: It is used in the production of silicon-based materials for medical devices and implants.
Industry: Trichlorosilane is a key raw material in the production of high-purity silicon for semiconductors and solar cells. .
Comparison with Similar Compounds
Trichlorosilane can be compared with other silicon hydrides and chlorosilanes:
Silane (SiH₄): A simpler silicon hydride used in the semiconductor industry.
Tetrachlorosilane (SiCl₄): A chlorosilane used in the production of fumed silica and as a precursor for silicon-based materials.
Dichlorosilane (H₂SiCl₂): Another chlorosilane used in the production of high-purity silicon and silicon-based compounds.
Uniqueness: Trichlorosilane is unique due to its combination of silicon, hydrogen, and chlorine atoms, which gives it distinct reactivity and versatility in various chemical processes. Its ability to serve as a precursor for high-purity silicon and its applications in both the semiconductor and solar industries highlight its importance .
Properties
InChI |
InChI=1S/Cl2Si/c1-3-2 | |
---|---|---|
Source | PubChem | |
URL | https://pubchem.ncbi.nlm.nih.gov | |
Description | Data deposited in or computed by PubChem | |
InChI Key |
BUMGIEFFCMBQDG-UHFFFAOYSA-N | |
Source | PubChem | |
URL | https://pubchem.ncbi.nlm.nih.gov | |
Description | Data deposited in or computed by PubChem | |
Canonical SMILES |
[Si](Cl)Cl | |
Source | PubChem | |
URL | https://pubchem.ncbi.nlm.nih.gov | |
Description | Data deposited in or computed by PubChem | |
Molecular Formula |
Cl2Si | |
Source | PubChem | |
URL | https://pubchem.ncbi.nlm.nih.gov | |
Description | Data deposited in or computed by PubChem | |
DSSTOX Substance ID |
DTXSID1052082 | |
Record name | Dichlorosilane | |
Source | EPA DSSTox | |
URL | https://comptox.epa.gov/dashboard/DTXSID1052082 | |
Description | DSSTox provides a high quality public chemistry resource for supporting improved predictive toxicology. | |
Molecular Weight |
98.99 g/mol | |
Source | PubChem | |
URL | https://pubchem.ncbi.nlm.nih.gov | |
Description | Data deposited in or computed by PubChem | |
CAS No. |
4109-96-0, 13569-32-9 | |
Record name | Dichlorosilane | |
Source | ChemIDplus | |
URL | https://pubchem.ncbi.nlm.nih.gov/substance/?source=chemidplus&sourceid=0004109960 | |
Description | ChemIDplus is a free, web search system that provides access to the structure and nomenclature authority files used for the identification of chemical substances cited in National Library of Medicine (NLM) databases, including the TOXNET system. | |
Record name | Dichlorosilylene | |
Source | ChemIDplus | |
URL | https://pubchem.ncbi.nlm.nih.gov/substance/?source=chemidplus&sourceid=0013569329 | |
Description | ChemIDplus is a free, web search system that provides access to the structure and nomenclature authority files used for the identification of chemical substances cited in National Library of Medicine (NLM) databases, including the TOXNET system. | |
Record name | Dichlorosilane | |
Source | EPA DSSTox | |
URL | https://comptox.epa.gov/dashboard/DTXSID1052082 | |
Description | DSSTox provides a high quality public chemistry resource for supporting improved predictive toxicology. | |
Record name | Dichlorosilane | |
Source | FDA Global Substance Registration System (GSRS) | |
URL | https://gsrs.ncats.nih.gov/ginas/app/beta/substances/WFA4RBW3D3 | |
Description | The FDA Global Substance Registration System (GSRS) enables the efficient and accurate exchange of information on what substances are in regulated products. Instead of relying on names, which vary across regulatory domains, countries, and regions, the GSRS knowledge base makes it possible for substances to be defined by standardized, scientific descriptions. | |
Explanation | Unless otherwise noted, the contents of the FDA website (www.fda.gov), both text and graphics, are not copyrighted. They are in the public domain and may be republished, reprinted and otherwise used freely by anyone without the need to obtain permission from FDA. Credit to the U.S. Food and Drug Administration as the source is appreciated but not required. | |
Disclaimer and Information on In-Vitro Research Products
Please be aware that all articles and product information presented on BenchChem are intended solely for informational purposes. The products available for purchase on BenchChem are specifically designed for in-vitro studies, which are conducted outside of living organisms. In-vitro studies, derived from the Latin term "in glass," involve experiments performed in controlled laboratory settings using cells or tissues. It is important to note that these products are not categorized as medicines or drugs, and they have not received approval from the FDA for the prevention, treatment, or cure of any medical condition, ailment, or disease. We must emphasize that any form of bodily introduction of these products into humans or animals is strictly prohibited by law. It is essential to adhere to these guidelines to ensure compliance with legal and ethical standards in research and experimentation.