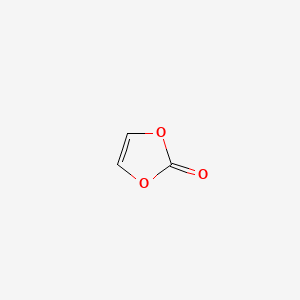
Vinylene carbonate
Overview
Description
Mechanism of Action
Target of Action
Vinylene carbonate (VC) is primarily used as an electrolyte additive in lithium-ion batteries . Its primary targets are the electrode surfaces within these batteries .
Mode of Action
VC interacts with its targets (the electrode surfaces) by forming a stable and protective Solid Electrolyte Interphase (SEI) film . This is achieved through a polymerization process, where VC forms a polymer of this compound . This SEI film greatly improves the electrochemical performance of the battery .
Biochemical Pathways
The formation of the SEI film involves a series of reduction reactions. VC commences reduction at less than 1.9 V, but the evolution of CO2 gas proceeds through a chemical step via a nucleophilic attack and VC ring opening . Additionally, VC scavenges H2O and reduced protons via hydrolysis and via proton abstraction from the carbon electrode to form ethylene carbonate .
Pharmacokinetics
While the term “pharmacokinetics” is typically used in the context of drug metabolism, in the case of VC, we can discuss its behavior in the battery environment. VC is known for its stability, which contributes to the longevity of the battery . It’s important to note that vc decomposes at temperatures above 80 °c .
Result of Action
The result of VC’s action is the formation of a more stable and protective SEI film at both electrode surfaces . This leads to improved electrochemical performance and reduced gas production in the battery, compared to cells without VC .
Action Environment
The action of VC is influenced by environmental factors such as temperature and the presence of water. VC reacts much more rapidly with water impurities, especially when in contact with hydroxides . This reaction forms products less likely to influence cell performance . Vc is thermolabile and decomposes at temperatures above 80 °c , which could impact its efficacy and stability.
Biochemical Analysis
Biochemical Properties
Vinylene carbonate plays a significant role in biochemical reactions due to its reactive double bond. It interacts with various enzymes, proteins, and other biomolecules. For instance, this compound can act as a dienophile in Diels-Alder reactions, facilitating the formation of complex cyclic structures. Additionally, it can undergo polymerization reactions, leading to the formation of poly(this compound), which has applications in medical and industrial fields .
Cellular Effects
This compound influences various types of cells and cellular processes. It has been observed to affect cell signaling pathways, gene expression, and cellular metabolism. For example, in the context of lithium-ion batteries, this compound is used as an additive to improve the performance of graphite electrodes. It helps in the formation of a stable solid electrolyte interphase (SEI) on the electrode surface, which enhances the overall efficiency and lifespan of the battery .
Molecular Mechanism
The molecular mechanism of this compound involves its interaction with biomolecules at the molecular level. This compound can undergo reduction and polymerization reactions, leading to the formation of a stable SEI on graphite electrodes in lithium-ion batteries. This SEI acts as a protective layer, preventing further degradation of the electrode material and improving the battery’s performance. Additionally, this compound can participate in Diels-Alder reactions and other cycloadditions, forming complex cyclic structures .
Dosage Effects in Animal Models
The effects of this compound can vary with different dosages in animal models. At low doses, this compound may exhibit beneficial effects, such as improving the performance of lithium-ion batteries. At high doses, it may cause toxic or adverse effects. For instance, excessive amounts of this compound could lead to the formation of unstable SEI layers, resulting in reduced battery performance and potential safety hazards .
Metabolic Pathways
This compound is involved in various metabolic pathways, interacting with enzymes and cofactors. In the context of lithium-ion batteries, this compound undergoes reduction and polymerization reactions to form a stable SEI on graphite electrodes. This SEI plays a crucial role in maintaining the battery’s performance by preventing further degradation of the electrode material. The specific metabolic pathways and interactions of this compound in other biochemical contexts require further exploration .
Transport and Distribution
This compound is transported and distributed within cells and tissues through various mechanisms. In lithium-ion batteries, this compound is added to the electrolyte solution, where it diffuses to the electrode surface and participates in the formation of the SEI. The transport and distribution of this compound in other biochemical contexts, such as its interaction with transporters or binding proteins, need to be studied in more detail .
Preparation Methods
Synthetic Routes and Reaction Conditions: Vinylene carbonate is commonly synthesized from ethylene carbonate. The process involves two main stages:
Photochlorination: Ethylene carbonate undergoes a UV-initiated photochlorination reaction with chlorine or sulfuryl chloride at 60-70°C to produce monochloroethylene carbonate.
Dehydrochlorination: The monochloroethylene carbonate is then dehydrochlorinated with a base such as triethylamine.
Industrial Production Methods: The industrial production of this compound involves optimizing the chlorination conditions to suppress the formation of by-products. The purification process is complex due to the thermolability of this compound, which decomposes at temperatures above 80°C. Techniques such as thin-film evaporation, fractional recrystallization, and zone melting are employed to obtain highly pure this compound .
Chemical Reactions Analysis
Vinylene carbonate undergoes various chemical reactions, including:
Polymerization: It can polymerize to form poly(this compound) or copolymerize with other monomers.
Decomposition: It decomposes to release carbon dioxide.
Ring-Opening Reactions: Under basic conditions, this compound undergoes ring-opening reactions, forming products that are less likely to influence cell performance in lithium-ion batteries.
Common Reagents and Conditions:
Polymerization: Radical initiators are commonly used.
Decomposition: Elevated temperatures or specific catalysts.
Ring-Opening Reactions: Bases such as hydroxides.
Major Products:
- Poly(this compound)
- Carbon dioxide
- Various ring-opened products
Scientific Research Applications
Vinylene carbonate has a wide range of applications in scientific research:
- Chemistry: Used as a monomer for homopolymerization and copolymerization, and as a dienophile in Diels-Alder reactions .
- Biology and Medicine: Employed in the synthesis of prodrugs such as olmesartan medoxomil and azilsartan medoxomil, where it serves as a cleavable group to release the active species .
- Industry: Widely used as an electrolyte additive in lithium-ion batteries to enhance performance and stability by forming a solid-electrolyte-interface (SEI) between the electrolyte and the negative electrode .
Comparison with Similar Compounds
- Ethylene carbonate
- Propylene carbonate
- Dimethyl carbonate
Vinylene carbonate’s ability to form a stable SEI and its reactivity as a monomer make it a valuable compound in various scientific and industrial applications.
Properties
IUPAC Name |
1,3-dioxol-2-one | |
---|---|---|
Source | PubChem | |
URL | https://pubchem.ncbi.nlm.nih.gov | |
Description | Data deposited in or computed by PubChem | |
InChI |
InChI=1S/C3H2O3/c4-3-5-1-2-6-3/h1-2H | |
Source | PubChem | |
URL | https://pubchem.ncbi.nlm.nih.gov | |
Description | Data deposited in or computed by PubChem | |
InChI Key |
VAYTZRYEBVHVLE-UHFFFAOYSA-N | |
Source | PubChem | |
URL | https://pubchem.ncbi.nlm.nih.gov | |
Description | Data deposited in or computed by PubChem | |
Canonical SMILES |
C1=COC(=O)O1 | |
Source | PubChem | |
URL | https://pubchem.ncbi.nlm.nih.gov | |
Description | Data deposited in or computed by PubChem | |
Molecular Formula |
C3H2O3 | |
Source | PubChem | |
URL | https://pubchem.ncbi.nlm.nih.gov | |
Description | Data deposited in or computed by PubChem | |
Related CAS |
25747-73-3 | |
Record name | Poly(vinylene carbonate) | |
Source | CAS Common Chemistry | |
URL | https://commonchemistry.cas.org/detail?cas_rn=25747-73-3 | |
Description | CAS Common Chemistry is an open community resource for accessing chemical information. Nearly 500,000 chemical substances from CAS REGISTRY cover areas of community interest, including common and frequently regulated chemicals, and those relevant to high school and undergraduate chemistry classes. This chemical information, curated by our expert scientists, is provided in alignment with our mission as a division of the American Chemical Society. | |
Explanation | The data from CAS Common Chemistry is provided under a CC-BY-NC 4.0 license, unless otherwise stated. | |
DSSTOX Substance ID |
DTXSID1074888 | |
Record name | 1,3-Dioxol-2-one | |
Source | EPA DSSTox | |
URL | https://comptox.epa.gov/dashboard/DTXSID1074888 | |
Description | DSSTox provides a high quality public chemistry resource for supporting improved predictive toxicology. | |
Molecular Weight |
86.05 g/mol | |
Source | PubChem | |
URL | https://pubchem.ncbi.nlm.nih.gov | |
Description | Data deposited in or computed by PubChem | |
Physical Description |
Liquid; Other Solid | |
Record name | 1,3-Dioxol-2-one | |
Source | EPA Chemicals under the TSCA | |
URL | https://www.epa.gov/chemicals-under-tsca | |
Description | EPA Chemicals under the Toxic Substances Control Act (TSCA) collection contains information on chemicals and their regulations under TSCA, including non-confidential content from the TSCA Chemical Substance Inventory and Chemical Data Reporting. | |
CAS No. |
872-36-6 | |
Record name | Vinylene carbonate | |
Source | CAS Common Chemistry | |
URL | https://commonchemistry.cas.org/detail?cas_rn=872-36-6 | |
Description | CAS Common Chemistry is an open community resource for accessing chemical information. Nearly 500,000 chemical substances from CAS REGISTRY cover areas of community interest, including common and frequently regulated chemicals, and those relevant to high school and undergraduate chemistry classes. This chemical information, curated by our expert scientists, is provided in alignment with our mission as a division of the American Chemical Society. | |
Explanation | The data from CAS Common Chemistry is provided under a CC-BY-NC 4.0 license, unless otherwise stated. | |
Record name | Vinylene carbonate | |
Source | ChemIDplus | |
URL | https://pubchem.ncbi.nlm.nih.gov/substance/?source=chemidplus&sourceid=0000872366 | |
Description | ChemIDplus is a free, web search system that provides access to the structure and nomenclature authority files used for the identification of chemical substances cited in National Library of Medicine (NLM) databases, including the TOXNET system. | |
Record name | VINYLENE CARBONATE | |
Source | DTP/NCI | |
URL | https://dtp.cancer.gov/dtpstandard/servlet/dwindex?searchtype=NSC&outputformat=html&searchlist=47201 | |
Description | The NCI Development Therapeutics Program (DTP) provides services and resources to the academic and private-sector research communities worldwide to facilitate the discovery and development of new cancer therapeutic agents. | |
Explanation | Unless otherwise indicated, all text within NCI products is free of copyright and may be reused without our permission. Credit the National Cancer Institute as the source. | |
Record name | VINYLENE CARBONATE | |
Source | DTP/NCI | |
URL | https://dtp.cancer.gov/dtpstandard/servlet/dwindex?searchtype=NSC&outputformat=html&searchlist=20980 | |
Description | The NCI Development Therapeutics Program (DTP) provides services and resources to the academic and private-sector research communities worldwide to facilitate the discovery and development of new cancer therapeutic agents. | |
Explanation | Unless otherwise indicated, all text within NCI products is free of copyright and may be reused without our permission. Credit the National Cancer Institute as the source. | |
Record name | 1,3-Dioxol-2-one | |
Source | EPA Chemicals under the TSCA | |
URL | https://www.epa.gov/chemicals-under-tsca | |
Description | EPA Chemicals under the Toxic Substances Control Act (TSCA) collection contains information on chemicals and their regulations under TSCA, including non-confidential content from the TSCA Chemical Substance Inventory and Chemical Data Reporting. | |
Record name | 1,3-Dioxol-2-one | |
Source | EPA DSSTox | |
URL | https://comptox.epa.gov/dashboard/DTXSID1074888 | |
Description | DSSTox provides a high quality public chemistry resource for supporting improved predictive toxicology. | |
Record name | Vinylene carbonate | |
Source | European Chemicals Agency (ECHA) | |
URL | https://echa.europa.eu/substance-information/-/substanceinfo/100.011.659 | |
Description | The European Chemicals Agency (ECHA) is an agency of the European Union which is the driving force among regulatory authorities in implementing the EU's groundbreaking chemicals legislation for the benefit of human health and the environment as well as for innovation and competitiveness. | |
Explanation | Use of the information, documents and data from the ECHA website is subject to the terms and conditions of this Legal Notice, and subject to other binding limitations provided for under applicable law, the information, documents and data made available on the ECHA website may be reproduced, distributed and/or used, totally or in part, for non-commercial purposes provided that ECHA is acknowledged as the source: "Source: European Chemicals Agency, http://echa.europa.eu/". Such acknowledgement must be included in each copy of the material. ECHA permits and encourages organisations and individuals to create links to the ECHA website under the following cumulative conditions: Links can only be made to webpages that provide a link to the Legal Notice page. | |
Record name | VINYLENE CARBONATE | |
Source | FDA Global Substance Registration System (GSRS) | |
URL | https://gsrs.ncats.nih.gov/ginas/app/beta/substances/1X0ZZF9WFV | |
Description | The FDA Global Substance Registration System (GSRS) enables the efficient and accurate exchange of information on what substances are in regulated products. Instead of relying on names, which vary across regulatory domains, countries, and regions, the GSRS knowledge base makes it possible for substances to be defined by standardized, scientific descriptions. | |
Explanation | Unless otherwise noted, the contents of the FDA website (www.fda.gov), both text and graphics, are not copyrighted. They are in the public domain and may be republished, reprinted and otherwise used freely by anyone without the need to obtain permission from FDA. Credit to the U.S. Food and Drug Administration as the source is appreciated but not required. | |
Synthesis routes and methods
Procedure details
Retrosynthesis Analysis
AI-Powered Synthesis Planning: Our tool employs the Template_relevance Pistachio, Template_relevance Bkms_metabolic, Template_relevance Pistachio_ringbreaker, Template_relevance Reaxys, Template_relevance Reaxys_biocatalysis model, leveraging a vast database of chemical reactions to predict feasible synthetic routes.
One-Step Synthesis Focus: Specifically designed for one-step synthesis, it provides concise and direct routes for your target compounds, streamlining the synthesis process.
Accurate Predictions: Utilizing the extensive PISTACHIO, BKMS_METABOLIC, PISTACHIO_RINGBREAKER, REAXYS, REAXYS_BIOCATALYSIS database, our tool offers high-accuracy predictions, reflecting the latest in chemical research and data.
Strategy Settings
Precursor scoring | Relevance Heuristic |
---|---|
Min. plausibility | 0.01 |
Model | Template_relevance |
Template Set | Pistachio/Bkms_metabolic/Pistachio_ringbreaker/Reaxys/Reaxys_biocatalysis |
Top-N result to add to graph | 6 |
Feasible Synthetic Routes
A: Vinylene carbonate enhances lithium-ion battery performance by forming a stable solid electrolyte interface (SEI) layer on the graphite anode. [, , ] This layer acts as a protective barrier, preventing further electrolyte decomposition and improving cycling efficiency by hindering side reactions. [, ]
A: At elevated temperatures, this compound forms a thermally stable layer on the cathode surface, particularly in batteries with high nickel content cathode materials. [] This layer, comprising polymeric species formed through reactions with ethylene carbonate, contributes to superior electrochemical and thermal behavior at higher temperatures. []
A: The molecular formula of this compound is C3H2O3, and its molecular weight is 86.05 g/mol. []
A: Yes, various spectroscopic techniques are employed to characterize this compound and its derivatives. These include Fourier Transform Infrared Spectroscopy (FTIR), Gas Chromatography-Mass Spectrometry (GC-MS), Nuclear Magnetic Resonance (NMR) spectroscopy, and X-ray Photoelectron Spectroscopy (XPS). [, , , ]
A: this compound improves electrolyte stability by scavenging water impurities, a critical factor in preserving the long-term performance of lithium-ion batteries. [] Its higher reactivity with water, especially in the presence of hydroxides, helps maintain the stability of the electrolyte solvent and salt. []
A: Yes, this compound positively affects antimony electrodes by increasing electrolyte stability and preventing the formation of a thick lithium fluoride (LiF) layer. [] This thinner layer allows for better contact between lithium and antimony, improving the reversibility of the electrochemical reaction and enhancing capacity retention during cycling. []
A: this compound acts as a versatile synthon in various transition metal-catalyzed C-H bond activation/cyclization reactions. [] It can serve as a surrogate for acetylene, ethynol, acetylation reagents, and other reactants. [, , , ] For instance, it enables the synthesis of fused dihydroisoquinoline frameworks through rhodium(III)-catalyzed annulation reactions. []
A: In reactions with amidines, this compound participates in a rhodium-catalyzed annulation process to produce 4-methylquinazolines. [] Interestingly, it functions as an acetylation reagent rather than an ethyne surrogate in this specific reaction. []
A: Density functional theory (DFT) calculations have been instrumental in elucidating the reductive decomposition mechanisms of this compound and ethylene carbonate in lithium-ion batteries. [] These calculations, incorporating solvent and salt effects, provide insights into the formation of SEI layer components and the superior performance of electrolytes containing this compound. []
A: DFT studies have been utilized to explore the mechanism of base-driven isomerization of exo-vinylene carbonates to endo-vinylene carbonates. [] These calculations address the complexities associated with charge separation steps, providing a deeper understanding of the reaction pathway. []
Disclaimer and Information on In-Vitro Research Products
Please be aware that all articles and product information presented on BenchChem are intended solely for informational purposes. The products available for purchase on BenchChem are specifically designed for in-vitro studies, which are conducted outside of living organisms. In-vitro studies, derived from the Latin term "in glass," involve experiments performed in controlled laboratory settings using cells or tissues. It is important to note that these products are not categorized as medicines or drugs, and they have not received approval from the FDA for the prevention, treatment, or cure of any medical condition, ailment, or disease. We must emphasize that any form of bodily introduction of these products into humans or animals is strictly prohibited by law. It is essential to adhere to these guidelines to ensure compliance with legal and ethical standards in research and experimentation.