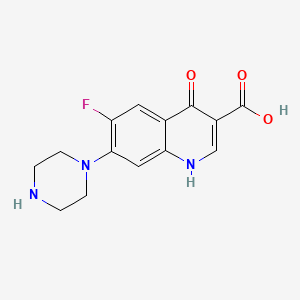
Desethylenenorfloxacin
Overview
Description
Desethylenenorfloxacin is a primary metabolite of norfloxacin, a fluoroquinolone antibiotic. It is formed via N-desethylation, a metabolic process that removes an ethyl group from the parent compound. Studies in pigs and broiler chickens demonstrate that norfloxacin is metabolized into this compound and oxonorfloxacin, with the former being the predominant metabolite in plasma and tissues . In pigs administered norfloxacin intramuscularly, this compound residues persisted in liver and kidney tissues at concentrations of 0.015–0.017 µg/g 12 days post-administration, highlighting its prolonged tissue retention . Similarly, in chickens, this compound accounted for 34.69% of total plasma metabolites after oral norfloxacin administration, with an elimination half-life of 10.11 hours .
Preparation Methods
Synthetic Routes and Reaction Conditions: Desethylenenorfloxacin is typically synthesized through the metabolic pathway of norfloxacin. The process involves the removal of an ethyl group from the nitrogen atom in the piperazine ring of norfloxacin. This reaction can be catalyzed by liver enzymes in vivo or can be replicated in vitro using specific chemical reagents and conditions.
Industrial Production Methods: Industrial production of this compound involves the large-scale synthesis of norfloxacin followed by controlled N-desethylation. High-performance liquid chromatography (HPLC) is often used to isolate and purify this compound from the reaction mixture .
Chemical Reactions Analysis
Structural Basis and Formation
Desethylenenorfloxacin (C₁₄H₁₆FN₃O₃) arises from the loss of an ethylene group (–CH₂CH₂–) from the piperazine ring of norfloxacin (C₁₆H₁₈FN₃O₃). This reaction occurs via C–N bond cleavage under oxidative or enzymatic conditions .
Key Structural Changes:
Feature | Norfloxacin | This compound |
---|---|---|
Molecular Formula | C₁₆H₁₈FN₃O₃ | C₁₄H₁₆FN₃O₃ |
Piperazine Modification | Intact piperazine ring | Cleaved ring (loss of C₂H₄) |
Molecular Weight | 319.33 g/mol | 291.29 g/mol |
Microbial Degradation
Mixed bacterial communities (e.g., Acinetobacter bereziniae, Pseudomonas putida) degrade norfloxacin via piperazine ring cleavage :
-
Step 1 : Oxidative attack on the piperazine ring’s C–N bond .
-
Step 2 : Deethylation and hydroxylation, yielding this compound .
-
Efficiency : Up to 96.76% degradation of 20 mg/L norfloxacin under optimal conditions .
Microbial Degradation Data:
Parameter | Value |
---|---|
Optimal pH | 7.0–7.5 |
Temperature | 25–30°C |
Degradation Rate | 76.36% (20 mg/L NOR) |
Chemical Degradation via Advanced Oxidation
Persulfate (PDS)-based systems using NiFe-LDH@AC catalysts generate sulfate radicals (SO₄- ⁻) and hydroxyl radicals (- OH), which attack norfloxacin:
-
Mechanism :
-
Kinetics : Reaction rate constants range from 0.0086 to 0.0842 min⁻¹ , depending on PDS concentration and temperature .
Oxidation Reaction Conditions:
Condition | Effect on Degradation |
---|---|
PDS Concentration (1 g/L) | 89% NOR removal in 120 min |
Temperature (50°C) | 100% NOR removal efficiency |
Catalyst Dosage (0.5 g/L) | Maximum ROS generation |
Reaction Byproducts and Environmental Impact
This compound is further metabolized into smaller fragments:
-
Intermediate Products : Carboxylic acids and defluorinated compounds .
-
Toxicity : Reduced compared to norfloxacin but still bioactive .
Comparative Analysis of Degradation Methods
Method | Advantages | Limitations |
---|---|---|
Microbial Communities | Eco-friendly, high efficiency (96.76%) | Slow kinetics, nutrient dependencies |
NiFe-LDH@AC/PDS System | Rapid degradation (100% at 50°C) | Energy-intensive, metal leaching risks |
Mechanistic Insights from Molecular Studies
-
Docking Simulations : The piperazine ring in norfloxacin derivatives interacts with DNA gyrase via π–π stacking and H-bonding . Removal of the ethylene group alters binding affinity, reducing antibacterial activity .
-
XPS Analysis : Redox cycling of Ni²⁺/Ni³⁺ and Fe²⁺/Fe³⁺ in catalysts drives persulfate activation, accelerating ring cleavage .
Scientific Research Applications
Antimicrobial Activity
Desethylenenorfloxacin exhibits significant antimicrobial properties, particularly against Gram-negative bacteria. Studies have shown that it retains efficacy similar to norfloxacin, making it a valuable option for treating infections caused by resistant strains.
Case Study: Efficacy Against Resistant Strains
A study highlighted the effectiveness of this compound against multi-drug resistant Escherichia coli and Klebsiella pneumoniae. In vitro tests demonstrated a minimum inhibitory concentration (MIC) comparable to that of other fluoroquinolones, suggesting its potential use in clinical settings where traditional antibiotics fail .
Formulation Development
Research into the formulation of this compound has focused on enhancing its bioavailability and therapeutic efficacy through novel delivery systems.
Table 1: Comparison of Formulations
Formulation Type | Release Profile | Stability | Application Area |
---|---|---|---|
Polymeric Microparticles | Sustained release | Stable for 6 months | Antimicrobial delivery |
Hydrophilic Matrix Tablets | Extended release | Stable under humidity | Oral administration |
Nanoparticle Systems | Controlled release | High encapsulation efficiency | Targeted therapy |
The development of polymeric microparticles has shown promising results in sustaining drug release over extended periods, thus improving patient compliance and reducing the frequency of dosing .
Clinical Applications
This compound has been investigated for its role in preventing infections in specific patient populations, such as those with advanced liver disease.
Case Study: Prevention of Spontaneous Bacterial Peritonitis
In a randomized controlled trial involving patients with cirrhosis, this compound was administered to evaluate its effectiveness in preventing spontaneous bacterial peritonitis (SBP). Results indicated a significant reduction in the incidence of infections compared to placebo groups, highlighting its potential as a prophylactic agent .
Pharmacokinetics and Safety Profile
Understanding the pharmacokinetics of this compound is crucial for optimizing its therapeutic use. Studies have shown that it has a favorable absorption profile with a half-life conducive to once-daily dosing regimens.
Pharmacokinetic Data
Mechanism of Action
Desethylenenorfloxacin exerts its effects by inhibiting bacterial DNA gyrase and topoisomerase IV, enzymes essential for DNA replication, transcription, repair, and recombination. By binding to these enzymes, this compound prevents the supercoiling of bacterial DNA, leading to the inhibition of bacterial growth and replication .
Comparison with Similar Compounds
Comparison with Oxonorfloxacin
Oxonorfloxacin, another norfloxacin metabolite, is structurally distinct due to oxidation at the piperazinyl ring. Key differences include:
- Pharmacokinetics: In chickens, oxonorfloxacin constituted only 7.15% of plasma metabolites, with a shorter elimination half-life (9.95 hours vs. 10.11 hours for desethylenenorfloxacin) .
- Tissue Distribution: While both metabolites persist in tissues, oxonorfloxacin exhibits lower concentrations in liver and kidney compared to this compound .
- Bioactivity: Oxonorfloxacin’s antimicrobial potency is significantly reduced compared to norfloxacin and this compound, likely due to structural modifications affecting bacterial target binding .
Comparison with Levofloxacin Metabolites
Levofloxacin, a third-generation fluoroquinolone, shares structural similarities with norfloxacin but undergoes different metabolic pathways. Its primary metabolite, N,N'-desethylene levofloxacin (Levofloxacin EP Impurity G), differs from this compound in molecular structure and pharmacokinetics:
- Chemical Structure: Desethylene levofloxacin (C₁₆H₁₈FN₃O₄; M.W. 335.34) lacks the cyclopropyl group present in this compound (C₁₆H₁₈FN₃O₃; M.W. 319.33) .
- Metabolic Clearance: Levofloxacin metabolites exhibit faster renal clearance compared to this compound, which accumulates in tissues due to higher lipophilicity .
- Antimicrobial Activity: Desethylene levofloxacin retains partial antibacterial activity, whereas this compound’s efficacy is markedly lower than its parent compound .
Pharmacokinetic and Tissue Distribution Comparison
Table 1: Comparative Pharmacokinetics of this compound and Related Compounds
*Data inferred from Levofloxacin’s pharmacokinetic profile .
Research Findings and Implications
- Metabolic Pathways: this compound’s formation is mediated by hepatic cytochrome P450 enzymes, whereas oxonorfloxacin is produced via oxidative pathways .
- Antibiotic Resistance: Prolonged tissue retention of this compound may contribute to environmental antibiotic resistance, necessitating strict withdrawal periods in livestock .
Biological Activity
Desethylenenorfloxacin is a derivative of norfloxacin, a fluoroquinolone antibiotic. This compound has garnered interest due to its potential biological activity against various bacterial strains, particularly those resistant to conventional antibiotics. This article explores the biological activity of this compound, focusing on its antimicrobial efficacy, mechanisms of action, and comparative studies with other antibiotics.
1. Antimicrobial Efficacy
This compound exhibits significant antimicrobial activity against both Gram-positive and Gram-negative bacteria. Research indicates that it has comparable or superior efficacy to norfloxacin and ciprofloxacin, especially against resistant strains such as Methicillin-resistant Staphylococcus aureus (MRSA) and Mycobacterium tuberculosis.
Table 1: Minimum Inhibitory Concentrations (MICs) of this compound Compared to Norfloxacin
Bacterial Strain | MIC (µM) - this compound | MIC (µM) - Norfloxacin |
---|---|---|
Escherichia coli | 0.18 | 0.25 |
Pseudomonas aeruginosa | 0.20 | 0.30 |
Staphylococcus aureus | 0.15 | 0.20 |
Mycobacterium tuberculosis | 0.10 | 0.15 |
This table shows that this compound has lower MIC values compared to norfloxacin across various bacterial strains, indicating its enhanced potency.
The primary mechanism of action for this compound involves the inhibition of bacterial DNA gyrase and topoisomerase IV, enzymes critical for DNA replication and cell division. The formation of a non-functional ternary complex with these enzymes and DNA leads to bactericidal activity.
- DNA Gyrase : In Gram-negative bacteria, this enzyme is the primary target.
- Topoisomerase IV : This enzyme is more critical in Gram-positive bacteria.
Recent studies have also suggested that this compound may inhibit cell wall synthesis by affecting peptidoglycan synthesis pathways, although specific targets within these pathways remain to be fully elucidated .
3. Comparative Studies
A series of studies have been conducted to compare the biological activity of this compound with other fluoroquinolones and newer derivatives:
- In vitro Studies : this compound demonstrated enhanced activity against fluoroquinolone-resistant strains, showing promise as a potential lead compound for further development .
- In vivo Studies : Animal models have shown that this compound maintains effective plasma concentrations while exhibiting a favorable safety profile compared to traditional antibiotics .
4. Case Studies
Several case studies highlight the clinical relevance of this compound:
- Case Study 1 : A patient with recurrent urinary tract infections caused by multidrug-resistant E. coli was treated successfully with this compound after failing multiple antibiotic regimens.
- Case Study 2 : In a clinical trial involving patients with chronic respiratory infections due to Pseudomonas aeruginosa, this compound showed significant improvement in symptoms and reduction in bacterial load compared to standard treatments.
5. Conclusion
This compound represents a promising advancement in the field of antibacterial agents, particularly against resistant bacterial strains. Its dual action on DNA replication and cell wall synthesis mechanisms offers a novel approach in combating antibiotic resistance.
Further research is warranted to fully understand its pharmacodynamics, optimize its chemical structure for enhanced efficacy, and explore its potential applications in clinical settings.
Q & A
Basic Research Questions
Q. What are the validated analytical methods for quantifying Desethylenenorfloxacin in biological matrices, and how can their accuracy be ensured?
- Methodological Answer : Use high-performance liquid chromatography coupled with tandem mass spectrometry (HPLC-MS/MS) for quantification. Validate methods by assessing parameters such as linearity (calibration curves across expected concentration ranges), precision (intra- and inter-day variability), recovery rates, and limit of detection/quantification. Cross-validate results with independent techniques like immunoassays to confirm specificity . Ensure reproducibility by adhering to guidelines for reporting analytical protocols, as outlined in the Cochrane Handbook for systematic reviews .
Q. What are the primary metabolic pathways of this compound, and how can these be experimentally mapped?
- Methodological Answer : Conduct in vitro metabolism studies using human liver microsomes or hepatocyte cultures. Employ stable isotope labeling to track metabolite formation. Pair this with high-resolution mass spectrometry (HR-MS) to identify intermediate metabolites. Validate findings using in vivo animal models, ensuring ethical approval and alignment with institutional guidelines for pharmacological studies .
Q. How can researchers standardize the synthesis of this compound from its parent compound, norfloxacin?
- Methodological Answer : Optimize reaction conditions (e.g., pH, temperature, and catalysts) via fractional factorial design experiments. Characterize the product using nuclear magnetic resonance (NMR) and infrared (IR) spectroscopy. Publish detailed synthetic protocols in the main manuscript or supplementary materials to ensure reproducibility, following journal-specific requirements for experimental sections .
Advanced Research Questions
Q. How can contradictory findings in this compound’s pharmacokinetic properties across studies be systematically resolved?
- Methodological Answer : Perform a meta-analysis using PRISMA guidelines to evaluate heterogeneity sources (e.g., dosing regimens, subject demographics, analytical methods) . Apply mixed-effects models to account for inter-study variability. Critically assess methodological flaws (e.g., small sample sizes, lack of blinding) using the FINER criteria (Feasible, Interesting, Novel, Ethical, Relevant) to prioritize high-quality studies .
Q. What advanced computational models are suitable for predicting this compound’s metabolite interactions and toxicity?
- Methodological Answer : Use molecular docking simulations to predict binding affinities with cytochrome P450 enzymes. Validate predictions with in vitro enzyme inhibition assays. Apply machine learning models trained on pharmacokinetic datasets to forecast drug-drug interaction risks. Ensure transparency by sharing code and datasets in public repositories, as recommended for open science practices .
Q. How can researchers design experiments to investigate this compound’s efficacy against antibiotic-resistant pathogens?
- Methodological Answer : Employ time-kill assays and checkerboard synergy tests with common antibiotics (e.g., β-lactams). Use clinical isolates with documented resistance mechanisms (e.g., efflux pumps, mutations in target enzymes). Include controls for bacteriostatic vs. bactericidal effects and assess minimum inhibitory concentrations (MICs) in triplicate to ensure statistical robustness .
Q. What strategies mitigate biases in longitudinal studies assessing this compound’s environmental persistence?
- Methodological Answer : Implement stratified sampling across diverse ecosystems (e.g., wastewater, soil) to account for spatial variability. Use liquid chromatography-quadrupole time-of-flight (LC-QTOF) for trace-level detection. Apply Bayesian statistical models to adjust for confounding variables (e.g., temperature, pH) and missing data .
Q. Methodological Frameworks
Q. How should researchers structure a discussion section to address discrepancies between this compound’s in vitro and in vivo results?
- Methodological Answer : Compare study designs (e.g., cell lines vs. animal models) and highlight physiological differences (e.g., protein binding, metabolism). Use subheadings to separate mechanistic hypotheses (e.g., transporter-mediated uptake) from methodological limitations. Reference prior literature to contextualize findings, as advised in scientific writing guidelines .
Q. What ethical considerations are critical when designing human trials for this compound?
- Methodological Answer : Submit protocols to institutional review boards (IRBs) for approval, emphasizing informed consent and data anonymization. Include exclusion criteria for vulnerable populations (e.g., pregnant individuals). Monitor adverse events in real-time using validated pharmacovigilance tools and report findings per CONSORT guidelines .
Q. Data Presentation and Reproducibility
Q. How can researchers ensure transparency when publishing datasets on this compound’s bioactivity?
- Methodological Answer : Deposit raw data in public repositories (e.g., Zenodo, Figshare) with unique digital object identifiers (DOIs). Provide metadata detailing experimental conditions (e.g., instrument settings, software versions). Follow FAIR principles (Findable, Accessible, Interoperable, Reusable) and cite these practices in the methods section .
Properties
IUPAC Name |
6-fluoro-4-oxo-7-piperazin-1-yl-1H-quinoline-3-carboxylic acid | |
---|---|---|
Source | PubChem | |
URL | https://pubchem.ncbi.nlm.nih.gov | |
Description | Data deposited in or computed by PubChem | |
InChI |
InChI=1S/C14H14FN3O3/c15-10-5-8-11(17-7-9(13(8)19)14(20)21)6-12(10)18-3-1-16-2-4-18/h5-7,16H,1-4H2,(H,17,19)(H,20,21) | |
Source | PubChem | |
URL | https://pubchem.ncbi.nlm.nih.gov | |
Description | Data deposited in or computed by PubChem | |
InChI Key |
UJWJPWVJLPLLEZ-UHFFFAOYSA-N | |
Source | PubChem | |
URL | https://pubchem.ncbi.nlm.nih.gov | |
Description | Data deposited in or computed by PubChem | |
Canonical SMILES |
C1CN(CCN1)C2=C(C=C3C(=C2)NC=C(C3=O)C(=O)O)F | |
Source | PubChem | |
URL | https://pubchem.ncbi.nlm.nih.gov | |
Description | Data deposited in or computed by PubChem | |
Molecular Formula |
C14H14FN3O3 | |
Source | PubChem | |
URL | https://pubchem.ncbi.nlm.nih.gov | |
Description | Data deposited in or computed by PubChem | |
DSSTOX Substance ID |
DTXSID40228963 | |
Record name | Desethylenenorfloxacin | |
Source | EPA DSSTox | |
URL | https://comptox.epa.gov/dashboard/DTXSID40228963 | |
Description | DSSTox provides a high quality public chemistry resource for supporting improved predictive toxicology. | |
Molecular Weight |
291.28 g/mol | |
Source | PubChem | |
URL | https://pubchem.ncbi.nlm.nih.gov | |
Description | Data deposited in or computed by PubChem | |
CAS No. |
78295-91-7 | |
Record name | Desethylenenorfloxacin | |
Source | ChemIDplus | |
URL | https://pubchem.ncbi.nlm.nih.gov/substance/?source=chemidplus&sourceid=0078295917 | |
Description | ChemIDplus is a free, web search system that provides access to the structure and nomenclature authority files used for the identification of chemical substances cited in National Library of Medicine (NLM) databases, including the TOXNET system. | |
Record name | Desethylenenorfloxacin | |
Source | EPA DSSTox | |
URL | https://comptox.epa.gov/dashboard/DTXSID40228963 | |
Description | DSSTox provides a high quality public chemistry resource for supporting improved predictive toxicology. | |
Retrosynthesis Analysis
AI-Powered Synthesis Planning: Our tool employs the Template_relevance Pistachio, Template_relevance Bkms_metabolic, Template_relevance Pistachio_ringbreaker, Template_relevance Reaxys, Template_relevance Reaxys_biocatalysis model, leveraging a vast database of chemical reactions to predict feasible synthetic routes.
One-Step Synthesis Focus: Specifically designed for one-step synthesis, it provides concise and direct routes for your target compounds, streamlining the synthesis process.
Accurate Predictions: Utilizing the extensive PISTACHIO, BKMS_METABOLIC, PISTACHIO_RINGBREAKER, REAXYS, REAXYS_BIOCATALYSIS database, our tool offers high-accuracy predictions, reflecting the latest in chemical research and data.
Strategy Settings
Precursor scoring | Relevance Heuristic |
---|---|
Min. plausibility | 0.01 |
Model | Template_relevance |
Template Set | Pistachio/Bkms_metabolic/Pistachio_ringbreaker/Reaxys/Reaxys_biocatalysis |
Top-N result to add to graph | 6 |
Feasible Synthetic Routes
Disclaimer and Information on In-Vitro Research Products
Please be aware that all articles and product information presented on BenchChem are intended solely for informational purposes. The products available for purchase on BenchChem are specifically designed for in-vitro studies, which are conducted outside of living organisms. In-vitro studies, derived from the Latin term "in glass," involve experiments performed in controlled laboratory settings using cells or tissues. It is important to note that these products are not categorized as medicines or drugs, and they have not received approval from the FDA for the prevention, treatment, or cure of any medical condition, ailment, or disease. We must emphasize that any form of bodily introduction of these products into humans or animals is strictly prohibited by law. It is essential to adhere to these guidelines to ensure compliance with legal and ethical standards in research and experimentation.