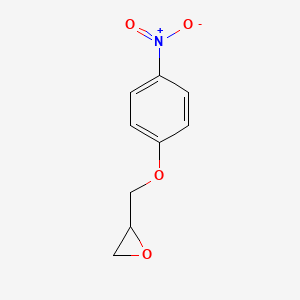
1,2-Epoxy-3-(p-nitrophenoxy)propane
Overview
Description
1,2-epoxy-3-(4-nitrophenoxy)propane is an epoxide that is oxirane substituted by a (4-nitrophenoxy)methyl group. It is an epoxide, a C-nitro compound and an aromatic ether. It derives from a 4-nitrophenol.
Mechanism of Action
Target of Action
The primary target of 1,2-epoxy-3-(4-nitrophenoxy)propane is the enzyme Glutathione S-transferase (GST) . This enzyme plays a crucial role in the detoxification of harmful compounds by conjugating them with glutathione .
Mode of Action
The interaction of 1,2-epoxy-3-(4-nitrophenoxy)propane with its target involves the nucleophilic attack of p-nitrophenol on propylene oxide, followed by the formation of an ether linkage between the two components . This reaction is facilitated by the GST enzyme, which acts on 1,2-epoxy-3-(4-nitrophenoxy)propane .
Biochemical Pathways
The action of 1,2-epoxy-3-(4-nitrophenoxy)propane affects the glutathione conjugation pathway . This pathway is responsible for the detoxification of a wide range of exogenous and endogenous hydrophobic electrophiles . The compound’s action on this pathway results in the formation of less harmful substances that can be easily excreted from the body.
Result of Action
The action of 1,2-epoxy-3-(4-nitrophenoxy)propane results in the detoxification of harmful compounds in the body . By facilitating their conjugation with glutathione, the compound helps in transforming these harmful substances into less toxic and more water-soluble metabolites that can be easily excreted from the body .
Action Environment
Environmental factors can influence the action, efficacy, and stability of 1,2-epoxy-3-(4-nitrophenoxy)propane. For instance, the presence of other chemicals in the environment can compete with the compound for the active site of GST, potentially affecting its efficacy . Moreover, factors such as temperature and pH can influence the stability of the compound.
Biochemical Analysis
Biochemical Properties
1,2-Epoxy-3-(p-nitrophenoxy)propane plays a crucial role in biochemical reactions, primarily as an inhibitor of certain enzymes. It has been shown to inhibit pepsin and other aspartic proteinases by reacting with the active site aspartic acid residues . The inhibition is irreversible and results from the esterification of essential carboxyl groups of the enzyme . This compound also interacts with other enzymes, such as low-molecular-weight phosphotyrosyl protein phosphatase and D-amino acid transferase . The nature of these interactions involves covalent binding to the active site residues, leading to the inactivation of the enzyme.
Cellular Effects
The effects of this compound on various types of cells and cellular processes are profound. This compound influences cell function by inhibiting key enzymes involved in cellular metabolism and signaling pathways. For instance, the inhibition of pepsin and other aspartic proteinases can disrupt protein digestion and processing in cells . Additionally, the compound’s interaction with phosphotyrosyl protein phosphatase can affect cell signaling pathways that rely on phosphorylation and dephosphorylation events . These interactions can lead to changes in gene expression and cellular metabolism, ultimately impacting cell function and viability.
Molecular Mechanism
The molecular mechanism of action of this compound involves its covalent binding to the active site residues of target enzymes. The epoxide group of the compound reacts with the carboxyl groups of aspartic acid residues in the enzyme’s active site, leading to irreversible inhibition . This esterification process prevents the enzyme from catalyzing its substrate, effectively inactivating it. The compound’s specificity for aspartic proteinases is due to the presence of these critical aspartic acid residues in their active sites . Additionally, the compound’s interaction with other enzymes, such as phosphotyrosyl protein phosphatase, involves similar covalent modifications that disrupt enzyme activity .
Temporal Effects in Laboratory Settings
In laboratory settings, the effects of this compound have been observed to change over time. The compound’s stability and degradation are critical factors that influence its long-term effects on cellular function. Studies have shown that the inhibition of enzymes by this compound is irreversible, leading to sustained inactivation of the target enzymes . The compound itself may undergo degradation over time, which can affect its potency and efficacy in long-term experiments . In vitro and in vivo studies have demonstrated that the compound’s inhibitory effects can persist for extended periods, depending on the stability of the compound and the experimental conditions .
Dosage Effects in Animal Models
The effects of this compound vary with different dosages in animal models. At low doses, the compound effectively inhibits target enzymes without causing significant toxicity . At higher doses, the compound can exhibit toxic effects, including cellular damage and disruption of normal physiological processes . Threshold effects have been observed, where a certain dosage level is required to achieve effective enzyme inhibition . Beyond this threshold, increasing the dosage can lead to adverse effects, highlighting the importance of dosage optimization in experimental settings .
Metabolic Pathways
This compound is involved in specific metabolic pathways, primarily through its interactions with enzymes and cofactors. The compound’s inhibition of aspartic proteinases and other enzymes can affect metabolic flux and metabolite levels in cells . For example, the inhibition of pepsin can disrupt protein digestion and processing, leading to changes in amino acid availability and utilization . Additionally, the compound’s interaction with phosphotyrosyl protein phosphatase can influence signaling pathways that regulate metabolic processes . These effects on metabolic pathways highlight the compound’s potential as a tool for studying cellular metabolism and enzyme regulation.
Transport and Distribution
The transport and distribution of this compound within cells and tissues are influenced by its interactions with transporters and binding proteins. The compound can be taken up by cells through passive diffusion or active transport mechanisms . Once inside the cell, it can interact with specific binding proteins that facilitate its localization and accumulation in certain cellular compartments . These interactions can affect the compound’s bioavailability and efficacy in inhibiting target enzymes . Additionally, the compound’s distribution within tissues can influence its overall impact on cellular function and metabolism .
Subcellular Localization
The subcellular localization of this compound is critical for its activity and function. The compound can be directed to specific compartments or organelles within the cell through targeting signals or post-translational modifications . For example, the compound’s interaction with aspartic proteinases may occur in lysosomes or other acidic compartments where these enzymes are active . The localization of the compound within these compartments can enhance its inhibitory effects and ensure its specificity for target enzymes . Additionally, the compound’s distribution within different subcellular compartments can influence its overall impact on cellular processes and functions .
Properties
IUPAC Name |
2-[(4-nitrophenoxy)methyl]oxirane | |
---|---|---|
Source | PubChem | |
URL | https://pubchem.ncbi.nlm.nih.gov | |
Description | Data deposited in or computed by PubChem | |
InChI |
InChI=1S/C9H9NO4/c11-10(12)7-1-3-8(4-2-7)13-5-9-6-14-9/h1-4,9H,5-6H2 | |
Source | PubChem | |
URL | https://pubchem.ncbi.nlm.nih.gov | |
Description | Data deposited in or computed by PubChem | |
InChI Key |
FPIGOBKNDYAZTP-UHFFFAOYSA-N | |
Source | PubChem | |
URL | https://pubchem.ncbi.nlm.nih.gov | |
Description | Data deposited in or computed by PubChem | |
Canonical SMILES |
C1C(O1)COC2=CC=C(C=C2)[N+](=O)[O-] | |
Source | PubChem | |
URL | https://pubchem.ncbi.nlm.nih.gov | |
Description | Data deposited in or computed by PubChem | |
Molecular Formula |
C9H9NO4 | |
Source | PubChem | |
URL | https://pubchem.ncbi.nlm.nih.gov | |
Description | Data deposited in or computed by PubChem | |
DSSTOX Substance ID |
DTXSID60863512 | |
Record name | Oxirane, 2-[(4-nitrophenoxy)methyl]- | |
Source | EPA DSSTox | |
URL | https://comptox.epa.gov/dashboard/DTXSID60863512 | |
Description | DSSTox provides a high quality public chemistry resource for supporting improved predictive toxicology. | |
Molecular Weight |
195.17 g/mol | |
Source | PubChem | |
URL | https://pubchem.ncbi.nlm.nih.gov | |
Description | Data deposited in or computed by PubChem | |
CAS No. |
5255-75-4 | |
Record name | 2-[(4-Nitrophenoxy)methyl]oxirane | |
Source | CAS Common Chemistry | |
URL | https://commonchemistry.cas.org/detail?cas_rn=5255-75-4 | |
Description | CAS Common Chemistry is an open community resource for accessing chemical information. Nearly 500,000 chemical substances from CAS REGISTRY cover areas of community interest, including common and frequently regulated chemicals, and those relevant to high school and undergraduate chemistry classes. This chemical information, curated by our expert scientists, is provided in alignment with our mission as a division of the American Chemical Society. | |
Explanation | The data from CAS Common Chemistry is provided under a CC-BY-NC 4.0 license, unless otherwise stated. | |
Record name | 1,2-Epoxy-3-(p-nitrophenoxy)propane | |
Source | ChemIDplus | |
URL | https://pubchem.ncbi.nlm.nih.gov/substance/?source=chemidplus&sourceid=0005255754 | |
Description | ChemIDplus is a free, web search system that provides access to the structure and nomenclature authority files used for the identification of chemical substances cited in National Library of Medicine (NLM) databases, including the TOXNET system. | |
Record name | Glycidyl 4-nitrophenyl ether | |
Source | DTP/NCI | |
URL | https://dtp.cancer.gov/dtpstandard/servlet/dwindex?searchtype=NSC&outputformat=html&searchlist=1733 | |
Description | The NCI Development Therapeutics Program (DTP) provides services and resources to the academic and private-sector research communities worldwide to facilitate the discovery and development of new cancer therapeutic agents. | |
Explanation | Unless otherwise indicated, all text within NCI products is free of copyright and may be reused without our permission. Credit the National Cancer Institute as the source. | |
Record name | Oxirane, 2-[(4-nitrophenoxy)methyl]- | |
Source | EPA Chemicals under the TSCA | |
URL | https://www.epa.gov/chemicals-under-tsca | |
Description | EPA Chemicals under the Toxic Substances Control Act (TSCA) collection contains information on chemicals and their regulations under TSCA, including non-confidential content from the TSCA Chemical Substance Inventory and Chemical Data Reporting. | |
Record name | Oxirane, 2-[(4-nitrophenoxy)methyl]- | |
Source | EPA DSSTox | |
URL | https://comptox.epa.gov/dashboard/DTXSID60863512 | |
Description | DSSTox provides a high quality public chemistry resource for supporting improved predictive toxicology. | |
Record name | [(p-nitrophenoxy)methyl]oxirane | |
Source | European Chemicals Agency (ECHA) | |
URL | https://echa.europa.eu/substance-information/-/substanceinfo/100.023.689 | |
Description | The European Chemicals Agency (ECHA) is an agency of the European Union which is the driving force among regulatory authorities in implementing the EU's groundbreaking chemicals legislation for the benefit of human health and the environment as well as for innovation and competitiveness. | |
Explanation | Use of the information, documents and data from the ECHA website is subject to the terms and conditions of this Legal Notice, and subject to other binding limitations provided for under applicable law, the information, documents and data made available on the ECHA website may be reproduced, distributed and/or used, totally or in part, for non-commercial purposes provided that ECHA is acknowledged as the source: "Source: European Chemicals Agency, http://echa.europa.eu/". Such acknowledgement must be included in each copy of the material. ECHA permits and encourages organisations and individuals to create links to the ECHA website under the following cumulative conditions: Links can only be made to webpages that provide a link to the Legal Notice page. | |
Record name | 1,2-EPOXY-3-(P-NITROPHENOXY)PROPANE | |
Source | FDA Global Substance Registration System (GSRS) | |
URL | https://gsrs.ncats.nih.gov/ginas/app/beta/substances/96071991U0 | |
Description | The FDA Global Substance Registration System (GSRS) enables the efficient and accurate exchange of information on what substances are in regulated products. Instead of relying on names, which vary across regulatory domains, countries, and regions, the GSRS knowledge base makes it possible for substances to be defined by standardized, scientific descriptions. | |
Explanation | Unless otherwise noted, the contents of the FDA website (www.fda.gov), both text and graphics, are not copyrighted. They are in the public domain and may be republished, reprinted and otherwise used freely by anyone without the need to obtain permission from FDA. Credit to the U.S. Food and Drug Administration as the source is appreciated but not required. | |
Synthesis routes and methods I
Procedure details
Synthesis routes and methods II
Procedure details
Synthesis routes and methods III
Procedure details
Synthesis routes and methods IV
Procedure details
Synthesis routes and methods V
Procedure details
Retrosynthesis Analysis
AI-Powered Synthesis Planning: Our tool employs the Template_relevance Pistachio, Template_relevance Bkms_metabolic, Template_relevance Pistachio_ringbreaker, Template_relevance Reaxys, Template_relevance Reaxys_biocatalysis model, leveraging a vast database of chemical reactions to predict feasible synthetic routes.
One-Step Synthesis Focus: Specifically designed for one-step synthesis, it provides concise and direct routes for your target compounds, streamlining the synthesis process.
Accurate Predictions: Utilizing the extensive PISTACHIO, BKMS_METABOLIC, PISTACHIO_RINGBREAKER, REAXYS, REAXYS_BIOCATALYSIS database, our tool offers high-accuracy predictions, reflecting the latest in chemical research and data.
Strategy Settings
Precursor scoring | Relevance Heuristic |
---|---|
Min. plausibility | 0.01 |
Model | Template_relevance |
Template Set | Pistachio/Bkms_metabolic/Pistachio_ringbreaker/Reaxys/Reaxys_biocatalysis |
Top-N result to add to graph | 6 |
Feasible Synthetic Routes
Q1: How does 1,2-Epoxy-3-(p-nitrophenoxy)propane interact with enzymes like Glutathione S-transferases (GSTs)?
A1: this compound acts as a substrate for certain GSTs [, , , , ]. This interaction involves the conjugation of glutathione to the epoxide ring of this compound, facilitating its detoxification and excretion.
Q2: What is the significance of this compound's interaction with Aspartic proteinases?
A2: this compound acts as an irreversible inhibitor of aspartic proteinases [, , , ]. It forms a covalent bond with the active site aspartic acid residues, leading to enzyme inactivation. This property has been investigated in the context of enzymes like pepsin, cathepsin D, and HIV protease.
Q3: Can you elaborate on the downstream effects of this compound's interaction with GSTs and aspartic proteinases?
A3: Inhibition of aspartic proteinases by this compound can disrupt various cellular processes, including protein degradation and processing []. This has implications for studying enzyme function and designing potential therapeutic inhibitors. On the other hand, its conjugation with glutathione by GSTs leads to detoxification and excretion, influencing the body's response to xenobiotics [, ].
Q4: What are the toxicological implications of this compound's interaction with DNA?
A4: Research has shown that this compound can covalently bind to guanine residues in DNA, leading to DNA adduct formation and exhibiting mutagenic activity in bacterial assays []. This highlights the compound's potential genotoxicity and warrants further investigation into its safety profile.
Q5: What is the molecular formula and weight of this compound?
A5: While the provided excerpts don't explicitly mention the molecular formula and weight, these can be derived from its chemical structure. The molecular formula is C9H9NO4 and its molecular weight is 195.17 g/mol.
Q6: Is there any spectroscopic data available for this compound?
A6: The excerpts provided do not delve into specific spectroscopic data like NMR or IR spectra for this compound. Further investigation of spectroscopic databases would be needed for this information.
Q7: What is known about the stability of this compound under various conditions?
A7: While the excerpts don't specifically address the compound's stability, its epoxide ring suggests sensitivity to hydrolysis, particularly under acidic or basic conditions. Specific studies would be needed to ascertain its stability profile.
Q8: Have any formulation strategies been explored to improve the stability, solubility, or bioavailability of this compound?
A8: The provided research excerpts do not mention specific formulation strategies for this compound. Given its potential applications as an enzyme inhibitor or research tool, investigating formulation approaches to enhance its delivery and efficacy could be beneficial.
Q9: What are the known catalytic properties of this compound?
A9: this compound itself does not possess catalytic properties. It acts as a substrate for enzymes like GSTs and as an irreversible inhibitor for aspartic proteinases, affecting their catalytic activities [, , , , ].
Q10: What are the applications of this compound in biochemical research?
A10: this compound serves as a valuable tool in biochemical research for:
- Studying GST enzyme activity and specificity [, , , , ].
- Investigating the structure and function of aspartic proteinases [, , , ].
- Developing and evaluating potential enzyme inhibitors, particularly for aspartic proteinases like HIV protease [].
Q11: What is the impact of structural modifications on the activity and potency of this compound analogs?
A11: Several research papers highlighted the synthesis and evaluation of this compound analogs with modifications to the epoxide ring and aromatic substituents [, ]. These modifications were shown to influence their reactivity and inhibitory potency toward HIV proteases. For instance, the presence of electron-withdrawing groups generally enhanced their inhibitory activity.
Q12: Has any research been conducted on the pharmacokinetic properties (ADME) of this compound?
A12: While the excerpts primarily focus on the in vitro activity of this compound, research has investigated its metabolism and excretion in rabbits and rats []. The studies revealed the formation of metabolites like 2-hydroxy-3-(p-nitrophenoxy)propionic acid and N-acetyl-S-[2-hydroxy-3-(p-nitrophenoxy)propyl]propyl]-L-cysteine, indicating its metabolic conversion and potential for excretion. Further studies are needed to comprehensively characterize its ADME profile.
Q13: What are the known toxicological effects of this compound?
A13: this compound has shown mutagenic activity and DNA adduct formation in laboratory studies [], suggesting potential genotoxicity. Additionally, administration of the compound to rats resulted in a marked decrease in hepatic GSH levels [], indicating potential liver toxicity. These findings warrant further investigation into its safety profile.
Q14: Are there specific SHE regulations governing the handling and use of this compound?
A14: While the provided excerpts don't explicitly mention specific SHE regulations, researchers should consult relevant safety data sheets and regulatory guidelines to ensure safe handling and disposal of this compound due to its potential genotoxicity and other potential hazards.
Q15: Have any computational studies been conducted on this compound, such as molecular modeling or QSAR analysis?
A15: The provided research snippets do not mention any specific computational studies on this compound. Given its use in studying enzyme activity and inhibition, computational approaches like molecular docking, molecular dynamics simulations, and QSAR studies could provide valuable insights into its interactions with target proteins and aid in the development of more potent and selective analogs.
Q16: Have any drug delivery systems been explored for this compound to enhance its targeting or delivery to specific tissues?
A16: The provided research excerpts don't discuss any specific drug delivery systems for this compound.
Q17: What analytical methods have been used to characterize and quantify this compound?
A17: Various analytical techniques have been employed in the studies, including:
- High-performance liquid chromatography (HPLC) for separating and identifying this compound and its metabolites [, ].
- Spectrophotometry for measuring enzyme activity by monitoring changes in absorbance associated with substrate consumption or product formation [, , , ].
- Mass spectrometry for identifying the site of this compound binding to proteins [].
Disclaimer and Information on In-Vitro Research Products
Please be aware that all articles and product information presented on BenchChem are intended solely for informational purposes. The products available for purchase on BenchChem are specifically designed for in-vitro studies, which are conducted outside of living organisms. In-vitro studies, derived from the Latin term "in glass," involve experiments performed in controlled laboratory settings using cells or tissues. It is important to note that these products are not categorized as medicines or drugs, and they have not received approval from the FDA for the prevention, treatment, or cure of any medical condition, ailment, or disease. We must emphasize that any form of bodily introduction of these products into humans or animals is strictly prohibited by law. It is essential to adhere to these guidelines to ensure compliance with legal and ethical standards in research and experimentation.