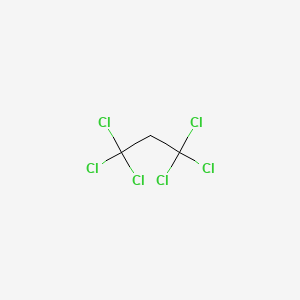
1,1,1,3,3,3-Hexachloropropane
Overview
Description
1,1,1,3,3,3-Hexachloropropane is a chemical compound composed of chlorine, hydrogen, and carbon, with the molecular formula C₃H₂Cl₆. It is a derivative of propane, where six hydrogen atoms are replaced by chlorine atoms on the terminal carbons. This compound is known for its high boiling point and significant density, making it a notable substance in various chemical processes .
Preparation Methods
Synthetic Routes and Reaction Conditions
1,1,1,3,3,3-Hexachloropropane can be synthesized through several methods:
Chlorination of Tetrachloropropane: The original synthesis by Davis and Whaley involved reacting 1,1,3,3-tetrachloropropane or 1,1,1,3-tetrachloropropane with chlorine at temperatures between 80-100°C.
Reaction of Carbon Tetrachloride and Dichloroethene: Another method involves reacting carbon tetrachloride (CCl₄) with 1,1-dichloroethene (Cl₂C=CH₂) at temperatures ranging from 80-150°C.
Industrial Production Methods
The industrial production of this compound typically follows the same synthetic routes mentioned above, with a focus on optimizing reaction conditions to maximize yield and purity. The use of continuous flow reactors and advanced catalytic systems can enhance the efficiency of these processes.
Chemical Reactions Analysis
1,1,1,3,3,3-Hexachloropropane undergoes various chemical reactions, including:
Substitution Reactions: This compound can participate in nucleophilic substitution reactions, where chlorine atoms are replaced by other nucleophiles. Common reagents include sodium hydroxide (NaOH) and potassium hydroxide (KOH).
Reduction Reactions: Reduction of this compound can be achieved using reducing agents such as lithium aluminum hydride (LiAlH₄), leading to the formation of less chlorinated derivatives.
Oxidation Reactions: Although less common, oxidation reactions can occur under specific conditions, potentially leading to the formation of chlorinated carboxylic acids.
Scientific Research Applications
1,1,1,3,3,3-Hexachloropropane has several applications in scientific research:
Biology and Medicine: Research has explored its effects on biological systems, including its impact on rat fetuses when inhaled at specific concentrations.
Industry: The compound is utilized in the production of various industrial chemicals and as a reagent in organic synthesis.
Mechanism of Action
The mechanism of action of 1,1,1,3,3,3-Hexachloropropane involves its interaction with nucleophiles and electrophiles in chemical reactions. The chlorine atoms on the terminal carbons make the molecule highly reactive, allowing it to participate in substitution and reduction reactions. The molecular targets and pathways involved depend on the specific reaction conditions and reagents used.
Comparison with Similar Compounds
1,1,1,3,3,3-Hexachloropropane can be compared with other similar compounds, such as:
1,1,2,2,3,3-Hexachloropropane: This compound has a different arrangement of chlorine atoms, leading to distinct chemical properties and reactivity.
1,1,1,3,3,3-Hexafluoropropane: A fluorinated analogue, used in various industrial applications.
Octachloropropane: Another chlorinated derivative with eight chlorine atoms, exhibiting unique chemical behavior.
The uniqueness of this compound lies in its specific chlorine substitution pattern, which influences its reactivity and applications in chemical synthesis.
Properties
IUPAC Name |
1,1,1,3,3,3-hexachloropropane | |
---|---|---|
Source | PubChem | |
URL | https://pubchem.ncbi.nlm.nih.gov | |
Description | Data deposited in or computed by PubChem | |
InChI |
InChI=1S/C3H2Cl6/c4-2(5,6)1-3(7,8)9/h1H2 | |
Source | PubChem | |
URL | https://pubchem.ncbi.nlm.nih.gov | |
Description | Data deposited in or computed by PubChem | |
InChI Key |
BBEAZDGZMVABIC-UHFFFAOYSA-N | |
Source | PubChem | |
URL | https://pubchem.ncbi.nlm.nih.gov | |
Description | Data deposited in or computed by PubChem | |
Canonical SMILES |
C(C(Cl)(Cl)Cl)C(Cl)(Cl)Cl | |
Source | PubChem | |
URL | https://pubchem.ncbi.nlm.nih.gov | |
Description | Data deposited in or computed by PubChem | |
Molecular Formula |
C3H2Cl6 | |
Source | PubChem | |
URL | https://pubchem.ncbi.nlm.nih.gov | |
Description | Data deposited in or computed by PubChem | |
DSSTOX Substance ID |
DTXSID3074600 | |
Record name | Propane, 1,1,1,3,3,3-hexachloro- | |
Source | EPA DSSTox | |
URL | https://comptox.epa.gov/dashboard/DTXSID3074600 | |
Description | DSSTox provides a high quality public chemistry resource for supporting improved predictive toxicology. | |
Molecular Weight |
250.8 g/mol | |
Source | PubChem | |
URL | https://pubchem.ncbi.nlm.nih.gov | |
Description | Data deposited in or computed by PubChem | |
CAS No. |
3607-78-1 | |
Record name | 1,1,1,3,3,3-Hexachloropropane | |
Source | CAS Common Chemistry | |
URL | https://commonchemistry.cas.org/detail?cas_rn=3607-78-1 | |
Description | CAS Common Chemistry is an open community resource for accessing chemical information. Nearly 500,000 chemical substances from CAS REGISTRY cover areas of community interest, including common and frequently regulated chemicals, and those relevant to high school and undergraduate chemistry classes. This chemical information, curated by our expert scientists, is provided in alignment with our mission as a division of the American Chemical Society. | |
Explanation | The data from CAS Common Chemistry is provided under a CC-BY-NC 4.0 license, unless otherwise stated. | |
Record name | 1,1,1,3,3,3-Hexachloropropane | |
Source | ChemIDplus | |
URL | https://pubchem.ncbi.nlm.nih.gov/substance/?source=chemidplus&sourceid=0003607781 | |
Description | ChemIDplus is a free, web search system that provides access to the structure and nomenclature authority files used for the identification of chemical substances cited in National Library of Medicine (NLM) databases, including the TOXNET system. | |
Record name | Propane, 1,1,1,3,3,3-hexachloro- | |
Source | EPA Chemicals under the TSCA | |
URL | https://www.epa.gov/chemicals-under-tsca | |
Description | EPA Chemicals under the Toxic Substances Control Act (TSCA) collection contains information on chemicals and their regulations under TSCA, including non-confidential content from the TSCA Chemical Substance Inventory and Chemical Data Reporting. | |
Record name | Propane, 1,1,1,3,3,3-hexachloro- | |
Source | EPA DSSTox | |
URL | https://comptox.epa.gov/dashboard/DTXSID3074600 | |
Description | DSSTox provides a high quality public chemistry resource for supporting improved predictive toxicology. | |
Record name | 1,1,1,3,3,3-hexachloropropane | |
Source | European Chemicals Agency (ECHA) | |
URL | https://echa.europa.eu/information-on-chemicals | |
Description | The European Chemicals Agency (ECHA) is an agency of the European Union which is the driving force among regulatory authorities in implementing the EU's groundbreaking chemicals legislation for the benefit of human health and the environment as well as for innovation and competitiveness. | |
Explanation | Use of the information, documents and data from the ECHA website is subject to the terms and conditions of this Legal Notice, and subject to other binding limitations provided for under applicable law, the information, documents and data made available on the ECHA website may be reproduced, distributed and/or used, totally or in part, for non-commercial purposes provided that ECHA is acknowledged as the source: "Source: European Chemicals Agency, http://echa.europa.eu/". Such acknowledgement must be included in each copy of the material. ECHA permits and encourages organisations and individuals to create links to the ECHA website under the following cumulative conditions: Links can only be made to webpages that provide a link to the Legal Notice page. | |
Synthesis routes and methods
Procedure details
Retrosynthesis Analysis
AI-Powered Synthesis Planning: Our tool employs the Template_relevance Pistachio, Template_relevance Bkms_metabolic, Template_relevance Pistachio_ringbreaker, Template_relevance Reaxys, Template_relevance Reaxys_biocatalysis model, leveraging a vast database of chemical reactions to predict feasible synthetic routes.
One-Step Synthesis Focus: Specifically designed for one-step synthesis, it provides concise and direct routes for your target compounds, streamlining the synthesis process.
Accurate Predictions: Utilizing the extensive PISTACHIO, BKMS_METABOLIC, PISTACHIO_RINGBREAKER, REAXYS, REAXYS_BIOCATALYSIS database, our tool offers high-accuracy predictions, reflecting the latest in chemical research and data.
Strategy Settings
Precursor scoring | Relevance Heuristic |
---|---|
Min. plausibility | 0.01 |
Model | Template_relevance |
Template Set | Pistachio/Bkms_metabolic/Pistachio_ringbreaker/Reaxys/Reaxys_biocatalysis |
Top-N result to add to graph | 6 |
Feasible Synthetic Routes
Disclaimer and Information on In-Vitro Research Products
Please be aware that all articles and product information presented on BenchChem are intended solely for informational purposes. The products available for purchase on BenchChem are specifically designed for in-vitro studies, which are conducted outside of living organisms. In-vitro studies, derived from the Latin term "in glass," involve experiments performed in controlled laboratory settings using cells or tissues. It is important to note that these products are not categorized as medicines or drugs, and they have not received approval from the FDA for the prevention, treatment, or cure of any medical condition, ailment, or disease. We must emphasize that any form of bodily introduction of these products into humans or animals is strictly prohibited by law. It is essential to adhere to these guidelines to ensure compliance with legal and ethical standards in research and experimentation.