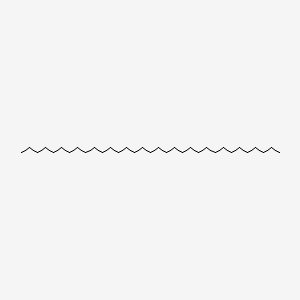
Tritriacontane
Overview
Description
Tritriacontane is a long-chain alkane consisting of an unbranched chain of 33 carbon atoms. It is a saturated hydrocarbon with the molecular formula C₃₃H₆₈. This compound is part of the paraffin series and is known for its high melting point and stability .
Biochemical Analysis
Biochemical Properties
Tritriacontane plays a role in biochemical reactions primarily as a structural component. It interacts with various enzymes and proteins involved in lipid metabolism. For instance, this compound can be metabolized by enzymes such as cytochrome P450 monooxygenases, which introduce oxygen into the hydrocarbon chain, leading to the formation of alcohols, aldehydes, and acids . These interactions are crucial for the compound’s integration into cellular lipid structures and its subsequent biochemical transformations.
Cellular Effects
This compound influences various cellular processes, including cell signaling pathways, gene expression, and cellular metabolism. It has been observed to affect the fluidity and permeability of cell membranes due to its hydrophobic nature . This can impact the function of membrane-bound proteins and receptors, thereby altering cell signaling pathways. Additionally, this compound can modulate gene expression by interacting with nuclear receptors that regulate lipid metabolism genes .
Molecular Mechanism
At the molecular level, this compound exerts its effects through binding interactions with biomolecules. It can integrate into lipid bilayers, affecting membrane dynamics and protein function . This compound may also inhibit or activate enzymes involved in lipid metabolism, such as lipases and desaturases, by altering the lipid environment in which these enzymes operate . Changes in gene expression can occur through the activation of transcription factors that respond to lipid-derived signals .
Temporal Effects in Laboratory Settings
In laboratory settings, the effects of this compound can change over time due to its stability and degradation. This compound is relatively stable under standard laboratory conditions, but it can undergo oxidation and other chemical transformations when exposed to light, heat, or reactive oxygen species . Long-term studies have shown that this compound can accumulate in cellular membranes, leading to alterations in membrane properties and cellular function over time .
Dosage Effects in Animal Models
The effects of this compound vary with different dosages in animal models. At low doses, this compound can be incorporated into lipid structures without causing significant toxicity . At high doses, it can lead to adverse effects such as lipid accumulation in tissues, disruption of cellular membranes, and oxidative stress . Threshold effects have been observed, where a certain dosage level is required to elicit noticeable biochemical and physiological changes .
Metabolic Pathways
This compound is involved in metabolic pathways related to lipid metabolism. It can be oxidized by cytochrome P450 enzymes to form various metabolites, including alcohols, aldehydes, and acids . These metabolites can further participate in metabolic pathways such as beta-oxidation, leading to the production of acetyl-CoA and other intermediates that enter the citric acid cycle . This compound’s metabolism can affect metabolic flux and the levels of various metabolites in cells .
Transport and Distribution
Within cells and tissues, this compound is transported and distributed through interactions with lipid transport proteins and binding proteins . It can be incorporated into lipoproteins for transport in the bloodstream and distributed to various tissues, including adipose tissue and the liver . This compound’s hydrophobic nature allows it to accumulate in lipid-rich environments, affecting its localization and function .
Subcellular Localization
This compound is primarily localized in cellular membranes and lipid droplets . Its subcellular localization is influenced by targeting signals and post-translational modifications that direct it to specific compartments or organelles . In membranes, this compound can affect membrane fluidity and the function of membrane-bound proteins . In lipid droplets, it can serve as a storage form of energy and a source of metabolic intermediates .
Preparation Methods
Synthetic Routes and Reaction Conditions: Tritriacontane can be synthesized through the catalytic hydrogenation of long-chain alkenes or alkynes. The process involves the use of hydrogen gas in the presence of a metal catalyst such as palladium, platinum, or nickel under high pressure and temperature conditions.
Industrial Production Methods: In industrial settings, this compound is often obtained from the fractional distillation of petroleum. The long-chain hydrocarbons are separated based on their boiling points, and this compound is isolated from the higher boiling fractions .
Types of Reactions:
Oxidation: this compound can undergo oxidation reactions, typically resulting in the formation of alcohols, aldehydes, or carboxylic acids. Common oxidizing agents include potassium permanganate and chromic acid.
Reduction: Although this compound is already a fully saturated hydrocarbon, it can be reduced to smaller alkanes through cracking processes.
Substitution: this compound can participate in substitution reactions, such as halogenation, where hydrogen atoms are replaced by halogen atoms (e.g., chlorine or bromine) in the presence of light or heat.
Common Reagents and Conditions:
Oxidation: Potassium permanganate (KMnO₄), chromic acid (H₂CrO₄)
Reduction: Hydrogen gas (H₂) with metal catalysts (e.g., palladium, platinum, nickel)
Substitution: Chlorine (Cl₂) or bromine (Br₂) with UV light or heat
Major Products Formed:
Oxidation: Alcohols, aldehydes, carboxylic acids
Reduction: Smaller alkanes
Substitution: Haloalkanes (e.g., trichlorotriacontane)
Scientific Research Applications
Tritriacontane has various applications in scientific research:
Chemistry: Used as a standard in gas chromatography for the calibration of retention times.
Biology: Studied for its role in the cuticular waxes of plants and insects, which provide protection and reduce water loss.
Medicine: Investigated for its potential use in drug delivery systems due to its hydrophobic nature.
Industry: Utilized in the production of lubricants, waxes, and coatings due to its high melting point and stability
Mechanism of Action
As a long-chain alkane, tritriacontane primarily exerts its effects through hydrophobic interactions. In biological systems, it can integrate into lipid bilayers, affecting membrane fluidity and permeability. Its hydrophobic nature also makes it an effective barrier in cuticular waxes, reducing water loss and providing protection against environmental stressors .
Comparison with Similar Compounds
- Eicosane (C₂₀H₄₂)
- Triacontane (C₃₀H₆₂)
- Dotriacontane (C₃₂H₆₆)
- Hentriacontane (C₃₁H₆₄)
Comparison: Tritriacontane is unique due to its longer carbon chain compared to eicosane, triacontane, and hentriacontane. This longer chain length contributes to its higher melting point and greater stability. Additionally, this compound’s hydrophobic nature and ability to form a protective barrier make it particularly useful in applications requiring high stability and low reactivity .
Properties
IUPAC Name |
tritriacontane | |
---|---|---|
Source | PubChem | |
URL | https://pubchem.ncbi.nlm.nih.gov | |
Description | Data deposited in or computed by PubChem | |
InChI |
InChI=1S/C33H68/c1-3-5-7-9-11-13-15-17-19-21-23-25-27-29-31-33-32-30-28-26-24-22-20-18-16-14-12-10-8-6-4-2/h3-33H2,1-2H3 | |
Source | PubChem | |
URL | https://pubchem.ncbi.nlm.nih.gov | |
Description | Data deposited in or computed by PubChem | |
InChI Key |
SUJUOAZFECLBOA-UHFFFAOYSA-N | |
Source | PubChem | |
URL | https://pubchem.ncbi.nlm.nih.gov | |
Description | Data deposited in or computed by PubChem | |
Canonical SMILES |
CCCCCCCCCCCCCCCCCCCCCCCCCCCCCCCCC | |
Source | PubChem | |
URL | https://pubchem.ncbi.nlm.nih.gov | |
Description | Data deposited in or computed by PubChem | |
Molecular Formula |
C33H68 | |
Source | PubChem | |
URL | https://pubchem.ncbi.nlm.nih.gov | |
Description | Data deposited in or computed by PubChem | |
DSSTOX Substance ID |
DTXSID5075444 | |
Record name | Tritriacontane | |
Source | EPA DSSTox | |
URL | https://comptox.epa.gov/dashboard/DTXSID5075444 | |
Description | DSSTox provides a high quality public chemistry resource for supporting improved predictive toxicology. | |
Molecular Weight |
464.9 g/mol | |
Source | PubChem | |
URL | https://pubchem.ncbi.nlm.nih.gov | |
Description | Data deposited in or computed by PubChem | |
Vapor Pressure |
4.02X10-11 mm Hg at 25 °C (Extrapolated) | |
Record name | n-Tritriacontane | |
Source | Hazardous Substances Data Bank (HSDB) | |
URL | https://pubchem.ncbi.nlm.nih.gov/source/hsdb/8363 | |
Description | The Hazardous Substances Data Bank (HSDB) is a toxicology database that focuses on the toxicology of potentially hazardous chemicals. It provides information on human exposure, industrial hygiene, emergency handling procedures, environmental fate, regulatory requirements, nanomaterials, and related areas. The information in HSDB has been assessed by a Scientific Review Panel. | |
Color/Form |
Orthorhombic crystals from ether, benzene | |
CAS No. |
630-05-7 | |
Record name | Tritriacontane | |
Source | CAS Common Chemistry | |
URL | https://commonchemistry.cas.org/detail?cas_rn=630-05-7 | |
Description | CAS Common Chemistry is an open community resource for accessing chemical information. Nearly 500,000 chemical substances from CAS REGISTRY cover areas of community interest, including common and frequently regulated chemicals, and those relevant to high school and undergraduate chemistry classes. This chemical information, curated by our expert scientists, is provided in alignment with our mission as a division of the American Chemical Society. | |
Explanation | The data from CAS Common Chemistry is provided under a CC-BY-NC 4.0 license, unless otherwise stated. | |
Record name | Tritriacontane | |
Source | ChemIDplus | |
URL | https://pubchem.ncbi.nlm.nih.gov/substance/?source=chemidplus&sourceid=0000630057 | |
Description | ChemIDplus is a free, web search system that provides access to the structure and nomenclature authority files used for the identification of chemical substances cited in National Library of Medicine (NLM) databases, including the TOXNET system. | |
Record name | Tritriacontane | |
Source | EPA DSSTox | |
URL | https://comptox.epa.gov/dashboard/DTXSID5075444 | |
Description | DSSTox provides a high quality public chemistry resource for supporting improved predictive toxicology. | |
Record name | Tritriacontane | |
Source | European Chemicals Agency (ECHA) | |
URL | https://echa.europa.eu/information-on-chemicals | |
Description | The European Chemicals Agency (ECHA) is an agency of the European Union which is the driving force among regulatory authorities in implementing the EU's groundbreaking chemicals legislation for the benefit of human health and the environment as well as for innovation and competitiveness. | |
Explanation | Use of the information, documents and data from the ECHA website is subject to the terms and conditions of this Legal Notice, and subject to other binding limitations provided for under applicable law, the information, documents and data made available on the ECHA website may be reproduced, distributed and/or used, totally or in part, for non-commercial purposes provided that ECHA is acknowledged as the source: "Source: European Chemicals Agency, http://echa.europa.eu/". Such acknowledgement must be included in each copy of the material. ECHA permits and encourages organisations and individuals to create links to the ECHA website under the following cumulative conditions: Links can only be made to webpages that provide a link to the Legal Notice page. | |
Record name | TRITRIACONTANE | |
Source | FDA Global Substance Registration System (GSRS) | |
URL | https://gsrs.ncats.nih.gov/ginas/app/beta/substances/4Y81Q52008 | |
Description | The FDA Global Substance Registration System (GSRS) enables the efficient and accurate exchange of information on what substances are in regulated products. Instead of relying on names, which vary across regulatory domains, countries, and regions, the GSRS knowledge base makes it possible for substances to be defined by standardized, scientific descriptions. | |
Explanation | Unless otherwise noted, the contents of the FDA website (www.fda.gov), both text and graphics, are not copyrighted. They are in the public domain and may be republished, reprinted and otherwise used freely by anyone without the need to obtain permission from FDA. Credit to the U.S. Food and Drug Administration as the source is appreciated but not required. | |
Record name | n-Tritriacontane | |
Source | Hazardous Substances Data Bank (HSDB) | |
URL | https://pubchem.ncbi.nlm.nih.gov/source/hsdb/8363 | |
Description | The Hazardous Substances Data Bank (HSDB) is a toxicology database that focuses on the toxicology of potentially hazardous chemicals. It provides information on human exposure, industrial hygiene, emergency handling procedures, environmental fate, regulatory requirements, nanomaterials, and related areas. The information in HSDB has been assessed by a Scientific Review Panel. | |
Melting Point |
71.2 °C | |
Record name | n-Tritriacontane | |
Source | Hazardous Substances Data Bank (HSDB) | |
URL | https://pubchem.ncbi.nlm.nih.gov/source/hsdb/8363 | |
Description | The Hazardous Substances Data Bank (HSDB) is a toxicology database that focuses on the toxicology of potentially hazardous chemicals. It provides information on human exposure, industrial hygiene, emergency handling procedures, environmental fate, regulatory requirements, nanomaterials, and related areas. The information in HSDB has been assessed by a Scientific Review Panel. | |
Q1: What is the molecular formula and weight of tritriacontane?
A1: this compound has the molecular formula C33H68 and a molecular weight of 464.92 g/mol.
Q2: What spectroscopic techniques are commonly used to characterize this compound?
A2: Gas Chromatography-Mass Spectrometry (GC-MS) [, , , , ] is frequently employed for identification and quantification. Nuclear Magnetic Resonance (NMR) spectroscopy (1H and 13C) [, , ] provides detailed structural information. X-ray diffraction [, , ] reveals its crystalline structure.
Q3: What are the characteristic peaks observed in the mass spectrum of this compound?
A3: The mass spectrum of this compound exhibits characteristic peaks corresponding to the molecular ion (M+) at m/z 464 and fragment ions arising from the cleavage of carbon-carbon bonds along the alkane chain.
Q4: Does the crystal structure of this compound change with temperature?
A4: Yes, this compound exhibits solid-solid phase transitions with temperature changes, which have been studied using techniques like SAXS [] and positron annihilation [].
Q5: How does the crystal structure of this compound influence its properties?
A5: The crystal structure of this compound significantly influences its physical properties such as melting point, hardness, and solubility. For instance, the tightly packed molecules in the crystalline state contribute to its high melting point.
Q6: Where is this compound found in nature?
A6: this compound is a common constituent of epicuticular waxes found on the surface of plant leaves and stems, contributing to their protection and water regulation [, , , , , ]. It is also found in propolis, a resinous mixture collected by bees [].
Q7: Can this compound be used as a marker in biological studies?
A7: Yes, this compound and other n-alkanes serve as valuable markers for studying various biological processes. For example, they can be used to estimate herbage intake and digestibility in animals [, , ], trace molecular exchange at solid-liquid interfaces [], and differentiate plant populations [, ].
Q8: What is the role of this compound in the epicuticular waxes of plants?
A8: As a component of epicuticular wax, this compound contributes to the plant's defense against water loss, UV radiation, and pathogen attack. Its presence can vary depending on the plant species and environmental conditions [, , ].
Q9: How does light quality influence the this compound content in tobacco leaves?
A9: Research suggests that specific light wavelengths can influence the accumulation of this compound and other cuticular extracts in tobacco leaves, highlighting the influence of light on plant wax biosynthesis [].
Q10: Can the presence of this compound in propolis indicate environmental contamination?
A10: The detection of hopanes, along with this compound, in propolis samples suggests bees may collect petroleum derivatives from asphalt, raising concerns about environmental contamination and its impact on propolis composition [].
Q11: Has this compound been synthesized?
A11: Yes, this compound has been synthesized []. Researchers have developed specific synthetic routes to produce this long-chain alkane, often utilizing readily available starting materials and established organic reactions.
Q12: Why was the synthesis of the levorotatory enantiomer of this compound significant?
A12: The synthesis of the levorotatory enantiomer of 4-methylthis compound helped researchers compare its properties to phthiocerane, a hydrocarbon derived from the tubercle bacillus, to understand the stereochemistry of the natural product [].
Disclaimer and Information on In-Vitro Research Products
Please be aware that all articles and product information presented on BenchChem are intended solely for informational purposes. The products available for purchase on BenchChem are specifically designed for in-vitro studies, which are conducted outside of living organisms. In-vitro studies, derived from the Latin term "in glass," involve experiments performed in controlled laboratory settings using cells or tissues. It is important to note that these products are not categorized as medicines or drugs, and they have not received approval from the FDA for the prevention, treatment, or cure of any medical condition, ailment, or disease. We must emphasize that any form of bodily introduction of these products into humans or animals is strictly prohibited by law. It is essential to adhere to these guidelines to ensure compliance with legal and ethical standards in research and experimentation.