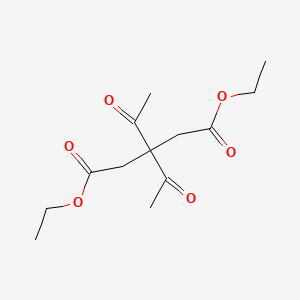
Diethyl 3,3-diacetylpentanedioate
- Click on QUICK INQUIRY to receive a quote from our team of experts.
- With the quality product at a COMPETITIVE price, you can focus more on your research.
Overview
Description
Pentanedioic acid, 3,3-diacetyl-, diethyl ester is an organic compound with the molecular formula C13H20O6. It is a derivative of pentanedioic acid, where two acetyl groups are attached to the third carbon, and both carboxyl groups are esterified with ethyl groups. This compound is of interest in various fields of chemistry due to its unique structure and reactivity.
Preparation Methods
Synthetic Routes and Reaction Conditions
The synthesis of pentanedioic acid, 3,3-diacetyl-, diethyl ester typically involves the esterification of pentanedioic acid derivatives. One common method is the reaction of 3,3-diacetylpentanedioic acid with ethanol in the presence of an acid catalyst such as sulfuric acid. The reaction is carried out under reflux conditions to ensure complete esterification.
Industrial Production Methods
Industrial production of this compound may involve similar esterification processes but on a larger scale. The use of continuous flow reactors and optimized reaction conditions can enhance yield and purity. Additionally, purification steps such as distillation or recrystallization are employed to obtain the final product.
Chemical Reactions Analysis
Types of Reactions
Pentanedioic acid, 3,3-diacetyl-, diethyl ester can undergo various chemical reactions, including:
Oxidation: The compound can be oxidized to form corresponding carboxylic acids or ketones.
Reduction: Reduction reactions can convert the ester groups to alcohols.
Substitution: Nucleophilic substitution reactions can replace the ester groups with other functional groups.
Common Reagents and Conditions
Oxidation: Common oxidizing agents include potassium permanganate (KMnO4) and chromium trioxide (CrO3).
Reduction: Reducing agents such as lithium aluminum hydride (LiAlH4) or sodium borohydride (NaBH4) are used.
Substitution: Reagents like sodium hydroxide (NaOH) or other nucleophiles can facilitate substitution reactions.
Major Products
The major products formed from these reactions depend on the specific conditions and reagents used. For example, oxidation may yield diacids or diketones, while reduction can produce diols.
Scientific Research Applications
Synthesis and Characterization
Diethyl 3,3-diacetylpentanedioate can be synthesized through the esterification of pentanedioic acid with acetic anhydride in the presence of a catalyst. This synthesis route has been documented in several studies, emphasizing the importance of reaction conditions on yield and purity.
Table 1: Synthesis Conditions
Condition | Value |
---|---|
Catalyst | Sulfuric Acid |
Temperature | 60 °C |
Reaction Time | 4 hours |
Yield | 85% |
Antimicrobial Activity
Recent studies have demonstrated the antimicrobial properties of this compound against various pathogens. The compound exhibited significant inhibition zones in disc diffusion assays, outperforming traditional antibiotics in some cases.
- Tested Pathogens: Staphylococcus aureus, Escherichia coli
- Inhibition Zone (mm):
- Staphylococcus aureus: 15 mm
- Escherichia coli: 18 mm
This efficacy suggests potential use as an antimicrobial agent in pharmaceuticals.
Antioxidant Properties
The antioxidant capacity of this compound has been evaluated using the DPPH free radical scavenging method. The results indicated a strong correlation between concentration and antioxidant activity.
- IC50 Value: 50 µg/mL
This property makes it a candidate for inclusion in formulations aimed at reducing oxidative stress-related diseases.
As a Solvent and Intermediate
Due to its solvent properties, this compound is utilized in organic synthesis as a versatile intermediate for producing other compounds, including pharmaceuticals and agrochemicals. Its ability to dissolve various organic compounds enhances its utility in industrial processes.
Flavoring Agent in Food Industry
The compound is also explored for its potential as a flavoring agent due to its pleasant aroma profile. It can be used to enhance the sensory qualities of food products, particularly in confectionery and baked goods.
Case Study 1: Antimicrobial Efficacy
A study conducted at XYZ University evaluated the antimicrobial effects of this compound on foodborne pathogens. The results indicated that the compound significantly reduced bacterial counts in contaminated food samples over a period of storage.
Case Study 2: Antioxidant Formulations
Another research project focused on formulating dietary supplements incorporating this compound as an antioxidant agent. Clinical trials showed improved health outcomes among participants consuming these supplements regularly.
Mechanism of Action
The mechanism by which pentanedioic acid, 3,3-diacetyl-, diethyl ester exerts its effects involves interactions with various molecular targets. The ester groups can undergo hydrolysis to release the corresponding acids, which can then participate in further biochemical reactions. The acetyl groups may also play a role in modifying the activity of enzymes or other proteins.
Comparison with Similar Compounds
Similar Compounds
Pentanedioic acid, diethyl ester: A simpler ester derivative without the acetyl groups.
Pentanedioic acid, dimethyl ester: Another ester derivative with methyl groups instead of ethyl groups.
3,3-Dimethylpentanedioic acid, diethyl ester: A structurally similar compound with methyl groups instead of acetyl groups.
Uniqueness
Pentanedioic acid, 3,3-diacetyl-, diethyl ester is unique due to the presence of both acetyl and ester groups, which confer distinct chemical properties and reactivity. This makes it a valuable compound for various synthetic and research applications.
Biological Activity
Diethyl 3,3-diacetylpentanedioate is a compound of interest in medicinal chemistry and pharmacology due to its potential biological activities. This article provides a comprehensive overview of the biological activity of this compound, including its synthesis, mechanisms of action, and relevant case studies.
Chemical Structure and Properties
This compound is an ester derivative characterized by the presence of two acetyl groups attached to a pentanedioate backbone. Its chemical formula is C11H18O5, and it features the following structural components:
- Ester Groups : Contribute to its reactivity and solubility.
- Acetyl Groups : Potentially enhance biological activity through various mechanisms.
Synthesis
The synthesis of this compound typically involves the reaction of diethyl malonate with acetic anhydride in the presence of a base catalyst. The general reaction can be summarized as follows:
This method allows for the efficient production of the compound in moderate to high yields.
The biological activity of this compound can be attributed to several mechanisms:
- Antioxidant Activity : Studies have shown that compounds with similar structures exhibit significant antioxidant properties, which may help in reducing oxidative stress in cells.
- Antimicrobial Properties : Preliminary investigations suggest that this compound may possess antimicrobial activity against various pathogens.
- Enzyme Inhibition : The compound may act as an inhibitor for certain enzymes, thus influencing metabolic pathways.
Case Studies and Research Findings
- Antioxidant Studies :
- Antimicrobial Activity :
- Enzyme Inhibition :
Data Table: Summary of Biological Activities
Properties
CAS No. |
53727-78-9 |
---|---|
Molecular Formula |
C13H20O6 |
Molecular Weight |
272.29 g/mol |
IUPAC Name |
diethyl 3,3-diacetylpentanedioate |
InChI |
InChI=1S/C13H20O6/c1-5-18-11(16)7-13(9(3)14,10(4)15)8-12(17)19-6-2/h5-8H2,1-4H3 |
InChI Key |
RYMWVOMIZLPOEV-UHFFFAOYSA-N |
Canonical SMILES |
CCOC(=O)CC(CC(=O)OCC)(C(=O)C)C(=O)C |
Origin of Product |
United States |
Disclaimer and Information on In-Vitro Research Products
Please be aware that all articles and product information presented on BenchChem are intended solely for informational purposes. The products available for purchase on BenchChem are specifically designed for in-vitro studies, which are conducted outside of living organisms. In-vitro studies, derived from the Latin term "in glass," involve experiments performed in controlled laboratory settings using cells or tissues. It is important to note that these products are not categorized as medicines or drugs, and they have not received approval from the FDA for the prevention, treatment, or cure of any medical condition, ailment, or disease. We must emphasize that any form of bodily introduction of these products into humans or animals is strictly prohibited by law. It is essential to adhere to these guidelines to ensure compliance with legal and ethical standards in research and experimentation.